What is soil fertility?
Soil fertility can be used to describe the availability of nutrients for plant uptake and in the broader context can be derived from soil type, soil organic matter status, soil properties including pH, as well as the concentration of available nutrients.
Soil fertility is usually reflective of land use. The fertility of a soil conveys not only the turnover of organic and inorganic inputs, but also the management of soil condition.
The conversion of natural vegetation to agricultural land uses changes soil processes and function to support a much less diversified but integral ecosystem.
Over time, soil fertility has become increasingly depleted in soil organic matter and nutrients, and inappropriate soil and plant management practices have often contributed to the development of constraints to sustainable production.
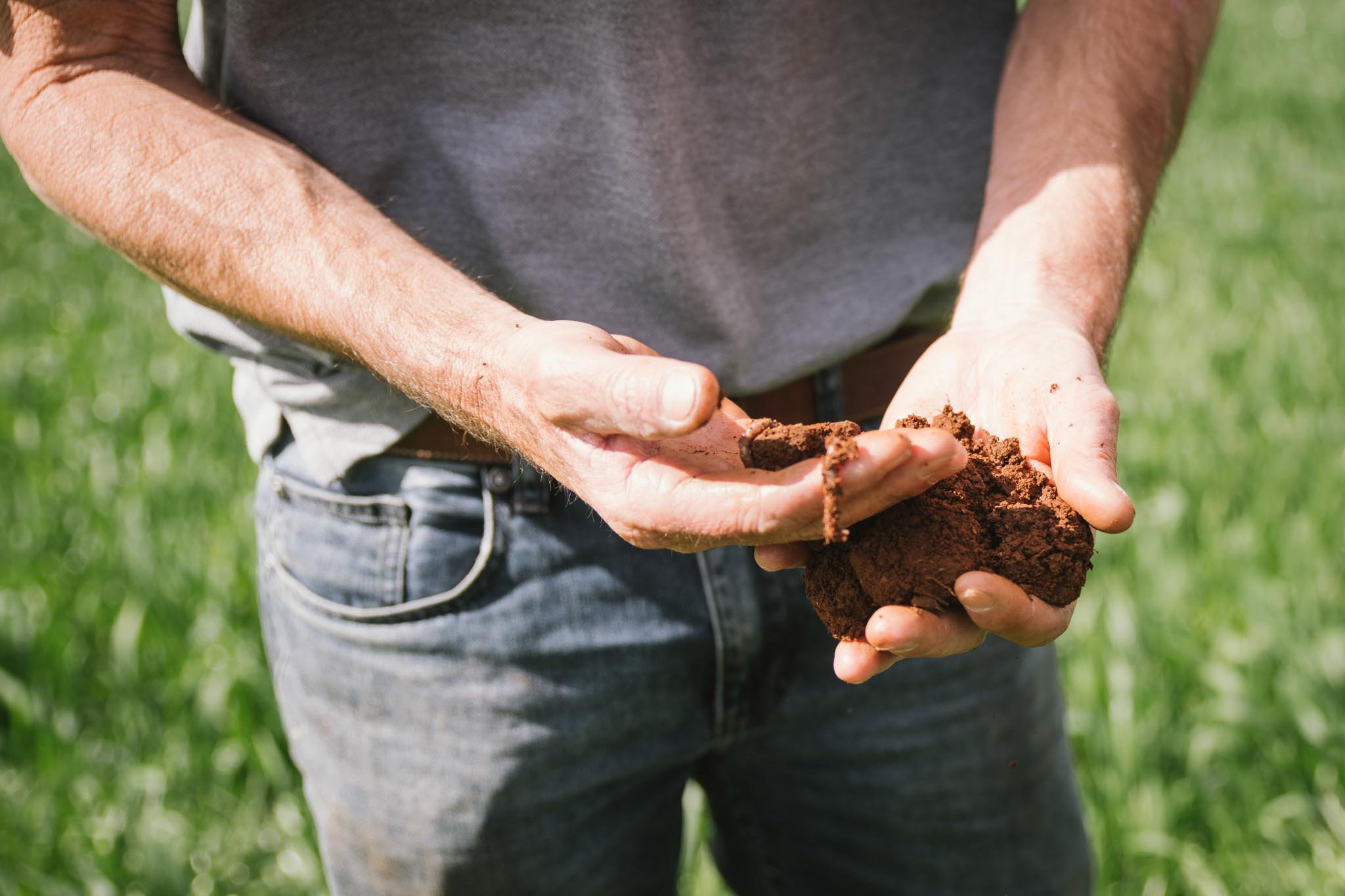
-
Optimum plant growth depends on balanced nutrition and the availability of all nutrients in sufficient quantities.
-
As soil fertility declines, organic matter input is reduced and soil structural degradation and soil erosion may increase.
-
Good nutrient management is necessary to maintain soil health and prevent land degradation.
-
Soil organic matter is a major storehouse of nutrients. Where soil organic matter has declined, so too will the ability of the soil to provide adequate nutrients for crops over the long-term.
-
A loss in soil fertility occurs when nutrient inputs are less than the removal of nutrients in agricultural products and by erosion and other means.
-
An effective nutrient management strategy involves integrating information from a variety of sources and formulating plans for nutrient additions to optimise crop production on a paddock- by-paddock and whole farm basis.
-
Nutrients are required in varying amounts and proportions during plant growth.
-
Efficient recycling of nutrients within the soil can offset the cost of inorganic fertiliser, reduce energy costs, cut waste, reduce potential environmental impacts and improve soil health.
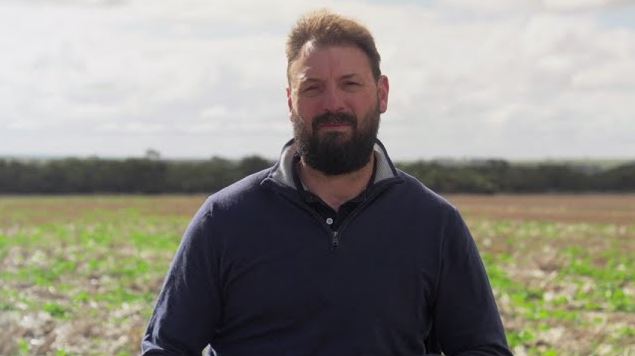
Nutrient management in farming systems in Western Australia
Nutrient management is a critical component of profitable farming systems in the south-western agricultural region of Western Australia. From: Soil Quality: 10 Plant Nutrition (Scanlan et al. 2023). Video: DPIRD and Red Empire.
What determines soil fertility?
Crop and pasture management techniques such as reduced cultivation, targeted fertiliser applications, pest and weed control, rotational grazing, and crop rotation diversity have contributed to large increases in crop yields and stocking rates. As a result, intensive cropping and grazing systems are often associated with the increased export of organic materials off-farm, a rundown in soil fertility (indicated by declining soil organic matter levels) and depleted soil nutrient reserves.
Why is soil fertility important?
Plants require carbon, oxygen and hydrogen which together make up 90 to 95% of the total plant biomass. Plants obtain carbon and oxygen primarily from the atmosphere. Hydrogen is obtained through water uptake and ionic forms from the soil.
Nutrients for plant growth
Plant nutrients are the chemical elements essential for plant growth and function.
There are 17 essential elements for all higher plants. The elements cobalt and sodium are essential for some classes of plants, but not all.
Relatively large amounts of macronutrients such as nitrogen (N), phosphorus (P), sulfur (S), potassium (K), calcium (Ca) and magnesium (Mg) are required for optimal plant growth.
Comparatively smaller amounts of trace nutrients (also known as micronutrients) such as copper (Cu), zinc (Zn), manganese (Mn), iron (Fe), molybdenum (Mo), boron (B) and chloride (Cl) are required.
General function of plant nutrients
Function | Nutrient(s) |
---|---|
Integral constituents of organic compounds | Nitrogen, sulfur |
Essential for energy acquisition and utilisation | Phosphorus |
Nutrients that are structurally associated with the cell wall | Calcium, boron, silicon |
Key parts of enzymes or of other essential entities of metabolism | Magnesium, iron, manganese, copper, molybdenum, nickel, zinc |
Activate or control that activity of enzymes | Potassium, sodium, chloride, magnesium, calcium, manganese, iron, zinc, copper |
Counter ions to positive or negative charges | Potassium, sodium, chloride, calcium, magnesium, nitrate, sulfate |
Non-specific functions that serve as major cellular osmotica | Potassium, sodium, chloride, nitrate, sulfate |
Citations
Functional role of nutrients Ma Q and Bell RW (2020) Chapter 7 Advances in understanding the potassium cycle in crop production. In: Rengel Z (Ed) Achieving sustainable crop nutrition. Burleigh Dodds Scientific Publishing, Cambridge UK. 27 pp. Available at: https://doi.org/10.19103/AS.2019.0062.09
Ma Q, Bell RW and Biddulph B (2019) Potassium application alleviates grain sterility and increases yield of wheat (Triticum aestivum) in frost-prone Mediterranean-type climate. Plant and Soil. 4: 203-216. Available at: https://doi.org/10.1007/s11104-018-3620-y
Ma Q, Bell RW, Scanlan C, Sarre G and Brennan RF (2015) Growth and yield responses in wheat and barley to potassium supply under drought or moderately saline conditions in south-western Australia. Crop and Pasture Science. 66(2): 135-144. Available at: https://doi.org/10.1071/CP14190
Prabhu AS, Fageria NK, Huber DM and Rodrigues FA (2007) Potassium and plant disease. In: Datnoff LE, Elmer WH and Huber DM (Eds) Mineral nutrition and plant disease. The American Phytopathological Society Press, Saint Paul, MN, pp. 57–78.
Organic and inorganic nutrients
The store of nutrients associated with soil organic matter is largely unavailable for direct plant uptake. A complex interaction of physical, chemical and biological processes is involved in converting rock minerals in organic materials to plant-available or ‘inorganic’ forms. This can involve physical weathering of mineral components and the mineralisation of organic fractions to plant-available cations and anions.
Currently, conventional farming systems rely predominantly on energy intensive inorganic fertilisers to supply nutrients due to ease of application and ability to time applications to plant demand.
However, increasingly organic wastes and manures as well as cover crops and natural forms of nitrogen fixation are being considered as a lower cost source of nutrients, with additional benefits in maintaining soil organic matter.
What are the impacts of plant nutrient deficiencies?
Deficiencies of any of the essential nutrients can decrease productivity by decreasing crop or pasture yield.
Manganese deficiency causes split seed in lupin. Deficiencies in calcium and boron commonly cause deformities in fruit that downgrade their marketability. Low concentrations of a range of nutrients can decrease seed vigour and hamper plant establishment.
Deficiencies of a range of nutrients increase the prevalence of leaf lesions due to foliar fungal disease. Root fungal infections can also increase with deficiencies of silicon, boron or manganese.
Nutrient deficiencies decrease nitrogen fixation by affecting nodule formation or nodule function when the requirements exceed those for growth of the host plant.
Whilst there are 17 essential elements for the growth of plants, for sustaining human life, in addition, there are another 33 essential nutrients comprising amino acids, lipids, vitamins, and a range of additional elements.
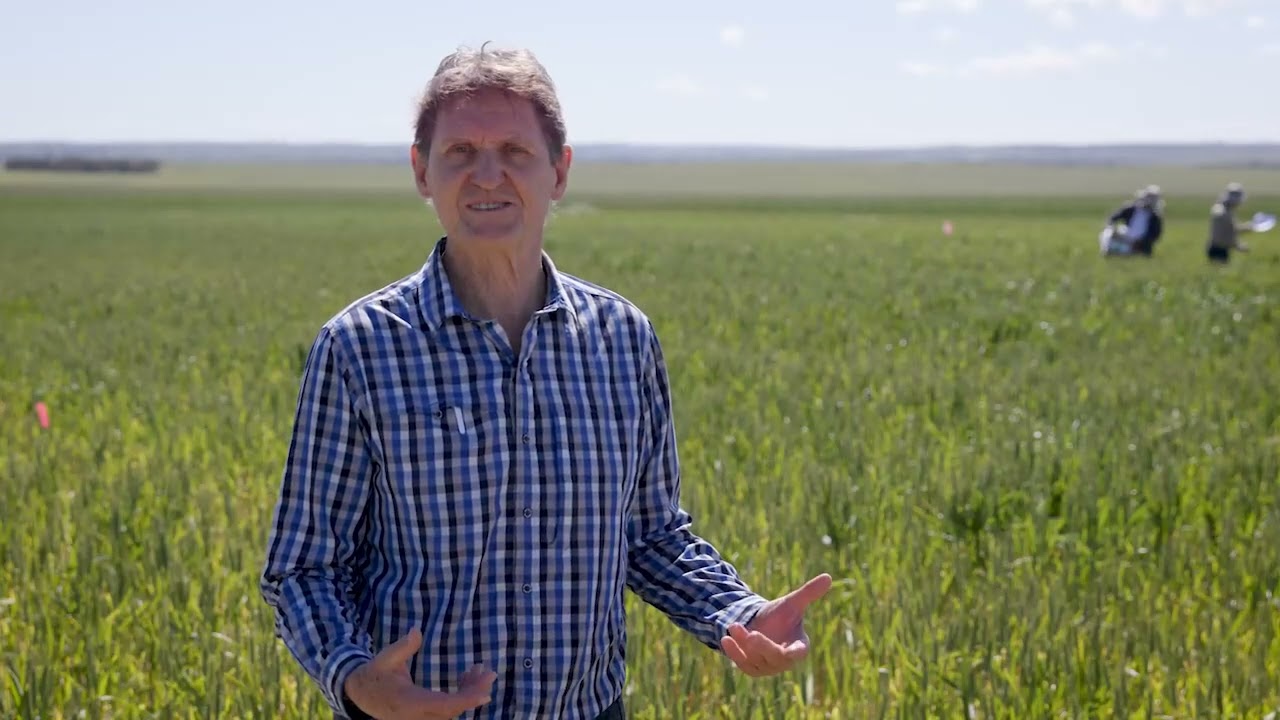
Role of potassium in tolerance to stress
Professor Richard Bell (Murdoch University) explains how potassium helps plants buffer against stress like disease and drought. From: Soil Quality: 10 Plant Nutrition (Scanlan et al. 2023).
Features of poor soil fertility
-
Low nutrient concentrations.
-
Low soil organic matter content.
-
Early plant senescence (‘haying off’ in cereals).
-
Small grains in grain crops.
-
Soil acidification.
-
Nutrient enrichment of waterways; known as eutrophication or algal blooms.
-
Poor plant growth and vigour.
Plant nutrient uptake
The majority of nutrients are taken up by plant roots from the soil solution (i.e. dissolved in water) although recent evidence shows that some nutrients may also be taken up in organic forms (such as amino acids).
Uptake by plant roots
The majority of the nutrients utilised by crop plants are taken up by roots. There are three processes by which plant roots acquire nutrients:
- Diffusion: a concentration gradient causes nutrients to move towards roots by diffusion. The concentration gradient is caused by depletion of nutrients in the rhizosphere around the root due to plant uptake.
- Mass flow: nutrients in the soil solution are drawn to the root surface by the movement of soil water to roots, driven by transpiration from leaves.
- Interception: the elongation of roots causes roots to contact soil particles with adsorbed nutrients.
Mass flow of nutrients to the root surface is the main plant uptake mechanism for nutrients that are mobile in the soil, such as nitrate, whereas diffusion is more important for nutrients that are immobile in the soil, for example, potassium and phosphate.
Plants are able to respond to low soil nutrient supply by increasing the rate of nutrient absorption by their roots. The roots of many plant species modify the chemical availability of nutrients in the rhizosphere through excretion of organic acids, enzymes, reductants, hydrogen ions (H+) or hydroxyl (OH–) ions. Roots may also synthesise more transporters and channels in membranes of cells to increase nutrient uptake rates. Most plants form a mycorrhizal symbiosis, particularly if soil phosphorus availability is low, which increases the uptake of nutrients that are mostly supplied by the diffusion pathway – phosphate, zinc and copper.
Other plant responses to increase the acquisition of nutrients from soil include:
- Increased allocation of resources, such as carbohydrates, to roots (under nitrogen and phosphorus deficiency)
- Changes in root architecture to exploit patches of higher nutrient availability in the root zone
- Increased root density for nutrient uptake of immobile elements, for example, cluster roots in lupin for phosphate uptake.
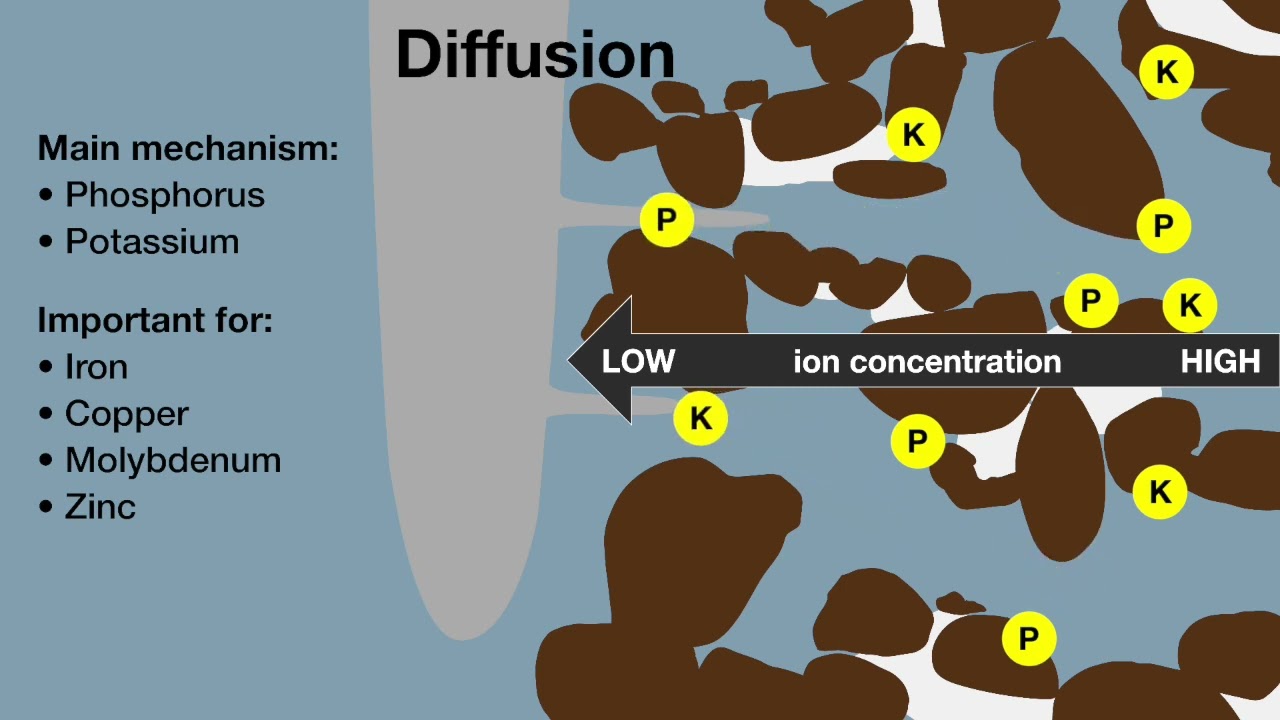
Processes of soil nutrient supply to plant roots.
Professor Richard Bell (Murdoch University) explains how plant roots take up nutrients from the soil by diffusion, mass flow, and interception. From Soil Quality: 10 Plant Nutrition (Scanlan et al. 2023). Concept by Gustavo Boitt, UWA. Animation: Science with Style.
Foliar uptake
Nutrient uptake by roots may be supplemented by foliar uptake either as a natural process or through application of liquid fertiliser solutions to the foliage. Foliar application of nutrients can be a very effective way of overcoming micronutrient deficiencies.
For macronutrients, foliar application can supplement uptake from soil or fertiliser. However, generally the amount that can be taken up is limited by scorching of the crop leaves when the nutrient concentration become too high in the foliar fertiliser.
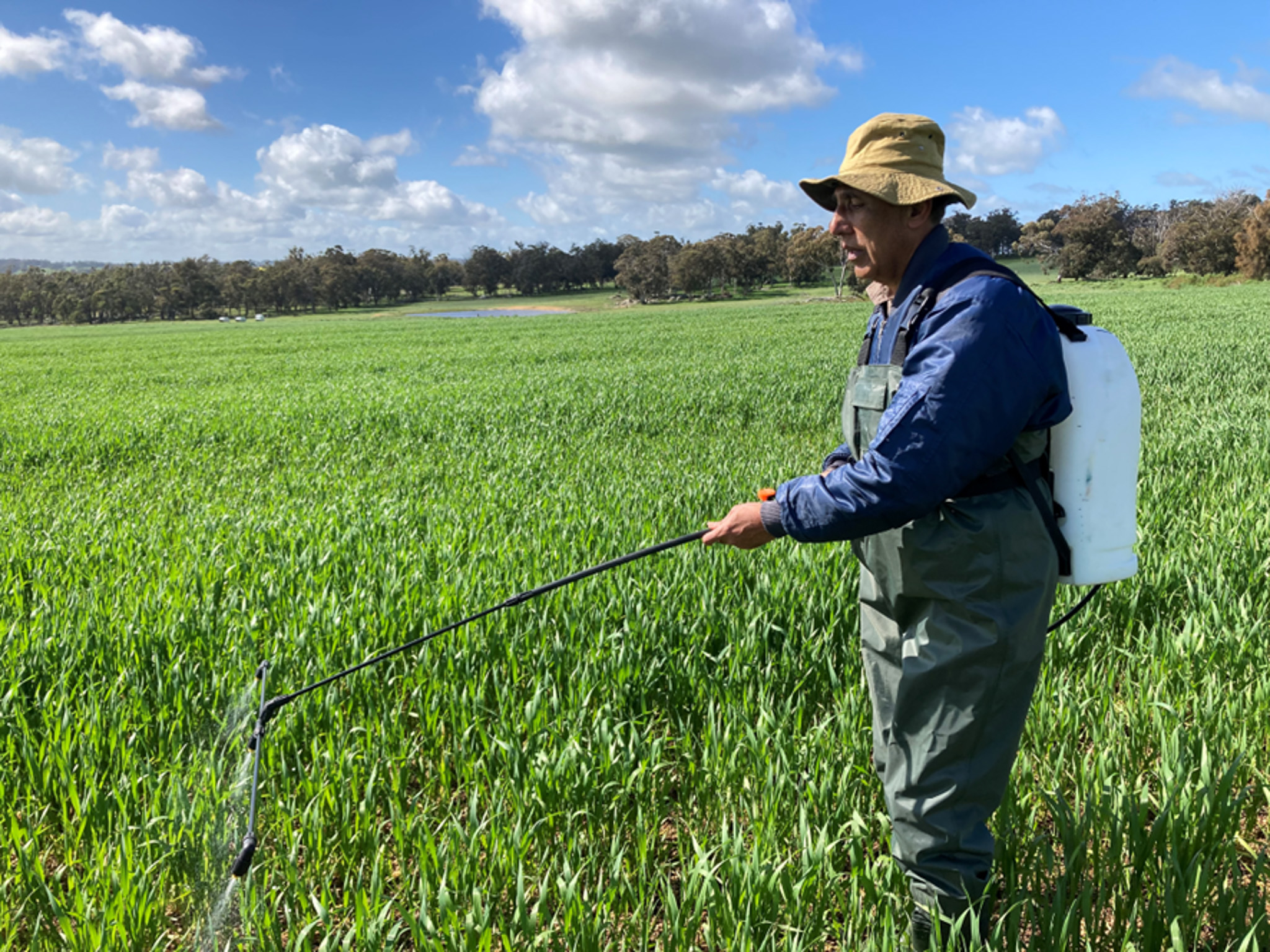
How does soil influence plant nutrition?
The ability of a plant to capture nutrients is partly influenced by:
- Root length
- Growth rate and physiological status
- Rooting pattern and distribution
- Nutrient demand
In turn, these plant features are influenced by a range of soil factors.
Coarse textured sandy soils rely heavily on their organic matter content for their ability to hold nutrients. As the capacity of these soils to protect organic matter is constrained, sands are commonly associated with leaching loss of mobile nutrients. However, where soil organic matter can be built up, micronutrients such as iron, copper, zinc, and manganese are tightly bound by the organic matter and prevented from leaching.
The capacity of a soil to hold nutrients influences yield response. Soils that adsorb nutrients strongly can render them unavailable to plants – low sorption capacity soils will reach a high concentration of nutrient in solution and are generally more fertiliser efficient, but are also more susceptible to leaching.
Soils high in iron and aluminium oxides (particularly those high in clay) adsorb phosphorus, molybdenum and sulphate. Similarly copper, zinc, and cobalt are adsorbed and may precipitate (solid separates from the solution rendering the nutrient immobile) under neutral to high pH conditions.
Soil pH is dependent on the type and concentration of cations in the soil solution (and is estimated by measuring hydrogen ions in solution). The pH buffering capacity is determined by the amount of adsorbed hydrogen ions the soil can adsorb because this determines the ability of a soil to resist changes in pH. Many light textured soils have low cation exchange capacity and low pH buffering capacity.
Soil pH alters the availability of nutrients. As pH increases iron, copper, zinc and manganese become less soluble and precipitate out of solution and become less available; whilst as pH decreases the availability of nitrogen, phosphorus, potassium, sulfur, calcium, molybdenum and magnesium is reduced.
Soil compaction constrains crop growth by limiting access to stored soil water and nutrients. In compacted soil, roots have to apply more force to displace soil particles when they are growing. As seminal roots reach the compacted layer, their rate of downward growth slows, because the roots encounter greater physical resistance in the compacted layer. As the seminal roots grow through the compacted layer, the rate of downward growth starts to increase again, but the impact on the root system is clear – it has less roots in the deeper layers and will have less access to subsoil water and nutrients later in the growing season.
Before starting a fertiliser program you need to consider your soil type, the existing pH and nutrient status, and the form and amount of fertiliser required to suit your farming situation. This information will help you to decide what type and quantity of nutrients you need.
The rule to remember with soluble fertilisers is to apply small amounts regularly, rather than a large amount occasionally. Plants can use only a fraction of any large amount applied, so most will be leached away or react with the soil (phosphorus, copper, zinc, etc). This is not only a waste of fertiliser and money, but it may also pollute ground water and surface waters and may contribute to the development of algal blooms. Organic fertilisers can be applied in larger amounts less often, as they release nutrients more slowly, but you still need to be careful how you use them.
Non-mobile nutrients such as phosphorus stay close to where they are applied, and are moved only by cultivation and seeding. Fertiliser placement should be used to ensure optimum plant uptake during growth and development, and phosphorus should be positioned where it is likely to be in moist soil for longer.
Yield responses also depend as much on the growing conditions and crop demand as they do on the level of extractable nutrient. The principles of soil fertility and plant nutrition may be equally applicable anywhere in the world, but in any given environment the response will vary. It is important to ensure that all nutrients required for plant growth are available in sufficient quantity as Liebig’s Law states that growth is controlled by the most limiting factor. Soils in Australia vary enormously in their ability to supply satisfactory nutrition to the crops and pastures growing on them. Many of our soils are acid (ammonium-nitrogen fertilisers make this problem worse) and low in calcium, magnesium, phosphorus and micronutrients.
High amounts of soil organic matter buffer the effect of fertilisers on pH, increase cation exchange capacity (CEC) and provide a source of nutrients. Different crop types have varying residue quality, with the largest variable being their nitrogen content. Legume crops fix nitrogen and generally result in increasing levels of soil nitrogen which benefit subsequent non-legume phases. These residues decompose more rapidly than cereals and release nitrogen throughout the subsequent growing season, but contribute less to building soil organic matter than more stable residues such as cereals and grasses. Plants such as capeweed and canola are good at scavenging for nitrogen and can increase the requirement for fertiliser nitrogen in subsequent crops.
The role of arbuscular mychorrizal (AM) fungi is considered beneficial to agricultural production in some instances. These organisms (and others) form a mutually beneficial (symbiotic) relationship with plant roots to actively help plants take up phosphorus through exploration of a greater volume of soil or mobilisation of bound nutrients, in exchange for plant sugars which provide energy for growth. The contribution of AM fungi to phosphorus uptake is comparatively greater in low phosphorus content soils than under high phosphorus fertiliser when AM colonisation is suppressed. Thus the contribution of AM fungi could be considered nominal under most practical agricultural conditions.
Soil temperature and moisture are rate limiting factors influencing biological activity, mineralisation of organic matter and the release of soil nutrients. Rainfall can also influence the location of nutrients in relation to rooting depth.
PAGE REFERENCES AND ACKNOWLEDGEMENTS
Material on this page adapted from:
- Hoyle FC (2007). Soil Health Knowledge Bank.
- Soil Quality ebook series. SoilsWest, Perth, Western Australia.
Last updated July 2024.