Soil water repellence overview
In Western Australia, 10.2 million hectares are at risk of soil water repellence. Of this total, 3.3 million hectares is of high risk and 6.9 million hectares of moderate risk. The estimated cost of production lost due to soil water repellence is $250–330 million per annum.
- Soil water repellence is widespread across the south-western agricultural region of Western Australia.
- Soil water repellence is often associated with sandy topsoil.
- Uneven wetting of the soil resulting from soil water repellence leads to poor emergence of crops and pastures and decreased productivity.
- The expression of water repellence has increased with greater use of dry sowing and minimum tillage farming systems as well as changes in rainfall patterns and intensity associated with climate change.
Sandy soil with low clay content is particularly prone to developing water repellence because the smaller surface area of the sand particles readily becomes saturated with waxy organic compounds, derived from plants and microbes. The occurrence and impact of soil water repellence can be increased by agricultural practices such as greater intensity of cropping, early and/or dry sowing, and minimum tillage. Minimum tillage results in greater stratification of organic matter at the soil surface. Water repellence is particularly a problem in low and medium rainfall environments (<500 mm annual rainfall).
The expression and impact of soil water repellence is increasing due to changes in the distribution and quantity of rainfall associated with climate change and more widespread use of early or dry sowing and minimum tillage farming systems.
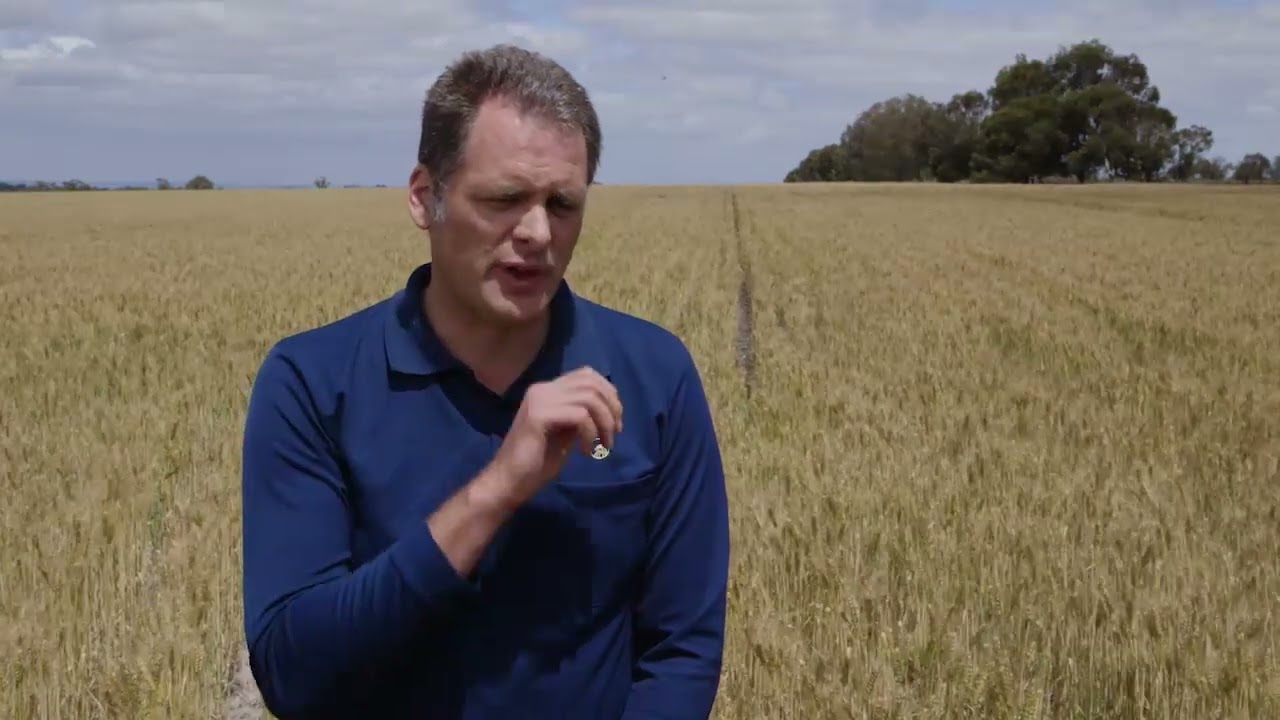
Soil water repellence: the essentials
This video provides an outline of the causes, impacts and management of soil water repellence. From Soil Quality: 7 Soil Water Repellence (Davies et al. 2022). Video talent: Stephen Davies, DPIRD; video production: Lomax Media.
Characteristics of water repellent soil
The risk of soil becoming water repellent is related to the size of soil particles and clay content as well as the presence of hydrophobic compounds. Soil particles with a low surface area are more readily saturated with hydrophobic material rendering them repellent.
Sandy textured soil with less than 10% clay is susceptible to water repellence and highly susceptible if the clay content is less than 5%. In south-eastern Australia, sandy soil commonly occurs as sandy dunes within dune-swale land systems while in Western Australia, larger areas of sandplain are more typical.
Soil with sandy loam texture and low clay content (around 10%) can also be repellent, though it is less common and typically less severe. Occasionally, finer textured soil can develop water repellence if the soil has an aggregated structure. In high rainfall environments (>1000 mm), soil with moderate to high clay content (>20%) can develop water repellence if large amounts of organic matter (>10%) are present.
Most commonly, water repellence occurs in the surface ‘organically-stained’ layers of sandy soil where the soil particles have a waxy coating of organic compounds. These compounds have both a hydrophobic and a hydrophilic end. They are strongly hydrophilic when wet. However, under drier conditions, the hydrophilic ends of the compounds bond strongly with each other and with sand particles leaving the hydrophobic ends exposed, resulting in the soil becoming water repellent. The severity of water repellence depends on the amount and type of hydrophobic compounds on the sand particles.
Prolonged wetting of soil can reverse water repellence. This may be because the bond between the organic compounds and the soil particles are weakened by the water, resulting in the compounds being displaced from the soil surface. Alternatively, organic compounds, such as humic or fulvic acid, may move into the soil water reducing the surface tension of the water allowing infiltration into the soil.
Reductions in water repellence may also be a result of microbial decomposition of waxes. Wax-decomposing bacteria have been isolated from both repellent and non-repellent soil and are active when the soil is wet.
Re-establishment of water repellence in soil after drying is not purely a factor of decreased soil moisture. In experiments, water repellence has not necessarily been restored by drying, but required heat as well to mobilise and to fuse new waxes onto sand particles. Field experiments have shown that repellence has a cyclic pattern with maximum expression of repellence at the end of dry, hot summer periods and least repellence during the wetter months of winter and spring. Expression of repellence has been shown to substantially increase if dry repellent soil is disturbed, which has implications for dry seeding and strategic tillage practices.
Although the severity of soil water repellence appears to have increased with drying climates, there is evidence of repellence in wetter climates too, particularly where organic matter loads are high.
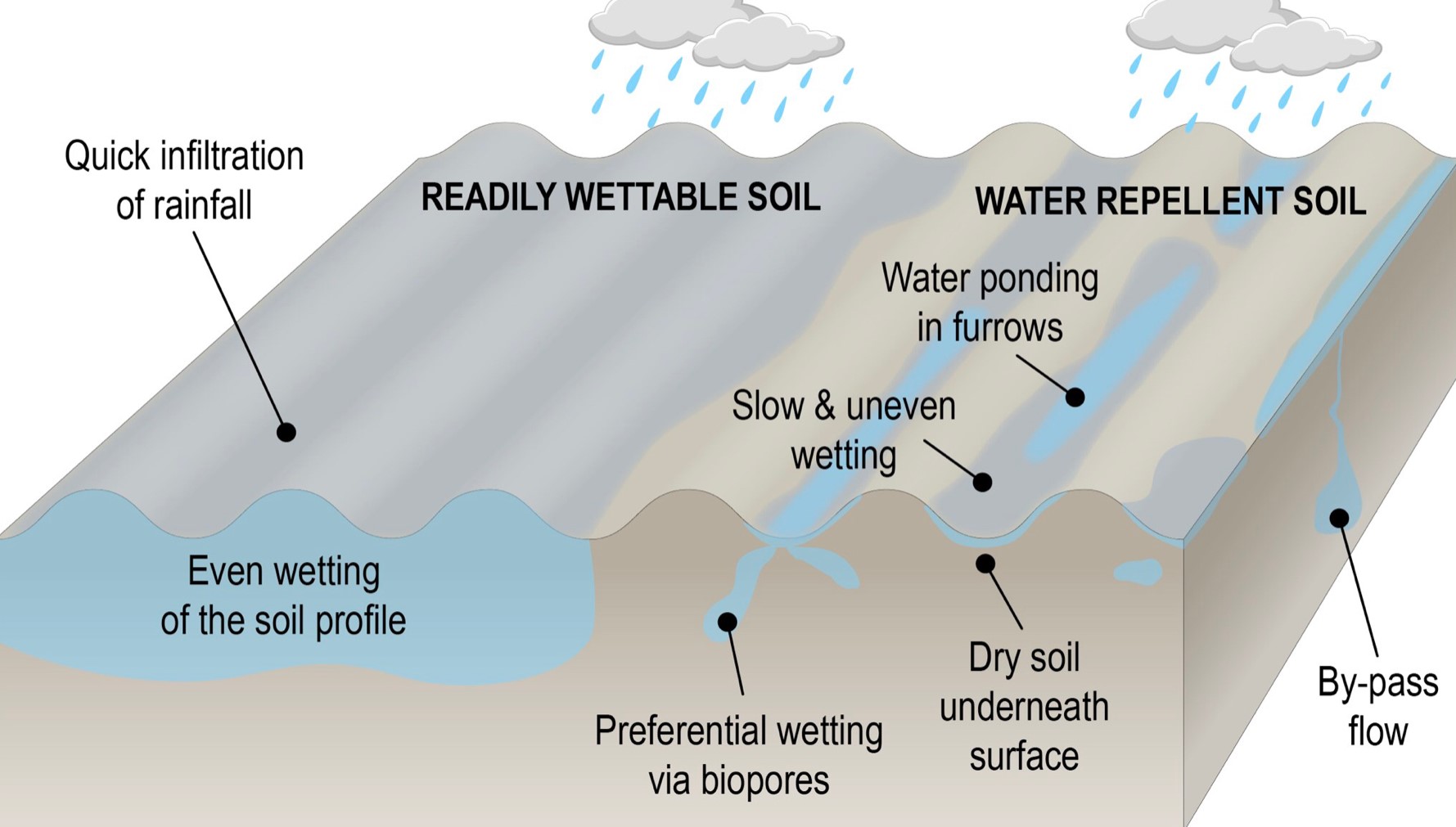
Causes of soil water repellence
Lipids and waxes from decomposing plant leaves, exudates from plant roots and some soil microbes are the sources of organic compounds that are most commonly responsible for water repellence.
Hydrophobic compounds may be derived from all plant species including native species. Legume crops and pastures are believed to produce higher amounts than other agricultural species, although there is some argument about which plants are most significant. In the south-western agricultural region of Western Australia, blue lupin (Lupinus cosentinii) has been associated with severe repellence. The impact of animal grazing and manure varies, although in Western Australia sheep camps have been anecdotally associated with a high incidence of water repellence. Sheep excrete alkanes and alkenes that increase the severity of water repellence.
In natural ecosystems, resins, waxes and aromatic oils produced by evergreen trees, temperate heathland and Mediterranean shrubland are commonly associated with soil water repellence. Fungal hyphae may also cause soil water repellence, but their influence is not entirely certain and seems to vary with species.
Some soil with 10–20% clay (up to more than 40%) may be water repellent under native vegetation. This is thought to occur as the clay forms aggregates, which reduce the surface area of the soil, or due to specific hydrophobic organic matter that coats the soil particles. Examples include soil associated with the mallet hills in the Great Southern, calcareous loams (also known as kopi soil) in the Zone of Ancient Drainage and loamy earth near Manjimup.
Water repellence below the surface of soil may develop following wildfire. Organic matter is vaporised in the fire then condenses and concentrates as it moves into deeper, cooler soil. This results in a water-repellent layer below the surface soil (around 50–100 mm). Temperatures exceeding 300°C are required for this to occur.
In agriculture, burning of plant residues is generally ‘cool’ and only exceeds 300°C for a short period of time. In this case organic matter may be consumed by the fire, which can reduce soil water repellence. However, other impacts can occur, such as increased susceptibility to erosion, loss of soil organic matter and of nutrients that are converted to gases during burning.
Impact of soil water repellence
Broad impacts
Soil water repellence impacts water movement into the soil and across the landscape. Poor and variable water infiltration and incomplete wetting of water repellent soil mean that water tends to enter the soil via preferred pathways, leaving other areas of the soil dry.
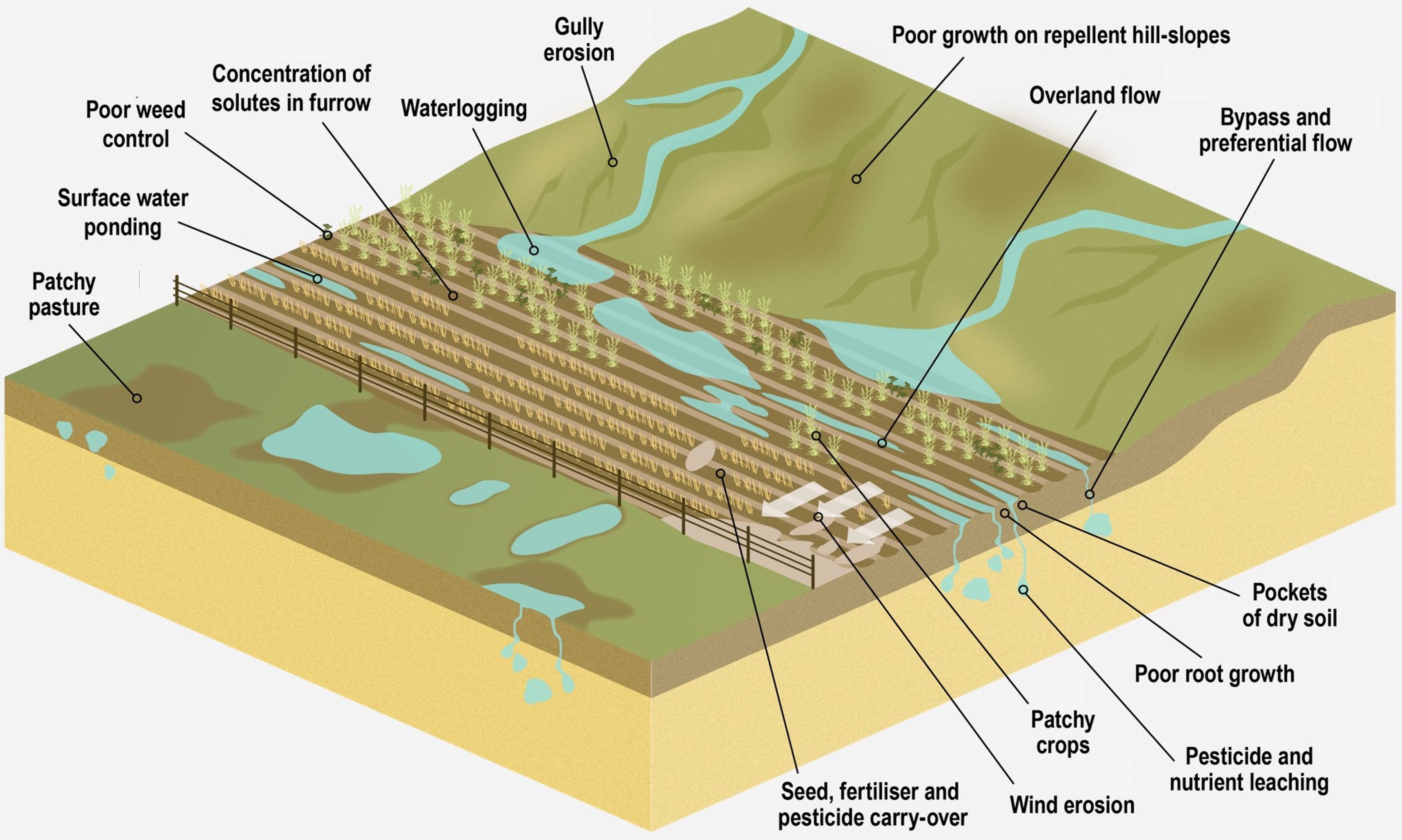
Soil water repellence may be viewed as an adaptation. In natural systems, it is an important mechanism for the selection and survival of native species of plants. In addition, water repellence is important for the structure of soil as the hydrophobic organic compounds enhance stability of aggregates. Reduced soil wettability decreases the access of microorganisms to organic matter, thereby ‘protecting’ it from turnover, further adding to aggregation of soil. These effects are particularly important in low rainfall environments.
These adaptations can provide benefits in agricultural systems as well. As with natural systems, organic matter associated with repellence adds to aggregation of soil particles (improved soil structure). Repellent topsoil can act as a mulch, decreasing evaporation. There can be increased water harvesting from repellent ridges into seeded furrows, even after small rainfall events. While these are real benefits, they are outweighed by the negative effects of poor soil wetting on the germination, emergence and yield of crops and pastures.
Expression in cropping systems
The transient nature of soil water repellence makes it a dynamic constraint, the expression of which varies both between seasons and within a season, depending on the timing and amount of rainfall and the temperature.
The expression of repellence is likely to increase in area and severity with the frequency of drier seasons and decreased intensity of autumn rainfall. This is exacerbated by responses in farming systems and practices to better manage drier seasons. For example, minimum tillage, increased frequency of dry seeding and the use of narrow knife points that can concentrate water repellent soil with seed.
The following factors affect the expression of soil water repellence in Western Australian cropping systems:
Expression of soil water repellence in the south-western agricultural region of Western Australia has worsened in association with increased frequency of years with a dry start to the growing season, with late and less frequent germinating rainfall events, often with less rainfall per event. These conditions lower the probability of the seed bed wetting up evenly over time.
Increased average daily temperatures can exacerbate the impact of marginal water conditions by increasing evaporation; in difficult-to-wet soil this can have an event greater impact.
Additionally, at high temperatures, there is increased fusion of the waxy compounds that cause repellency onto soil surfaces.
There has been extensive adoption of minimum tillage and stubble retention cropping systems in Western Australia (nearly 90% of growers). The concentration of soil organic matter at the soil surface has increased with these practices, resulting in more severe expression of soil water repellence. The waxes that cause water repellence are a component of the organic matter that is concentrated at the soil surface, where soil moisture conditions and lack of disturbance limit the opportunity for microbial breakdown of these compounds. While all plant residues contribute to soil water repellence, some such as legumes, may contain more of the waxes that cause water repellence.
More frequent dry and early seeding, before repellent soils have had time to wet up, worsens the expression of soil water repellence and exacerbates staggered crop germination and establishment.
In addition, the physical disturbance of soil when seeding dry can increase the expression of soil water repellence. Dry seeding where knife points are used can result in repellent topsoil that flows around the point and concentrates in the furrow and in close proximity with the seed, adversely impacting wetting and germination of the seed.
In water repellent soil, narrow knife points allow dry repellent topsoil to readily flow around the point and into the furrow, concentrating it with the seed and fertiliser. This results in poor wetting of the soil around the seed, inhibiting germination and dissolution of fertiliser. Widespread use of knife points has resulted in greater expression of soil water repellence.
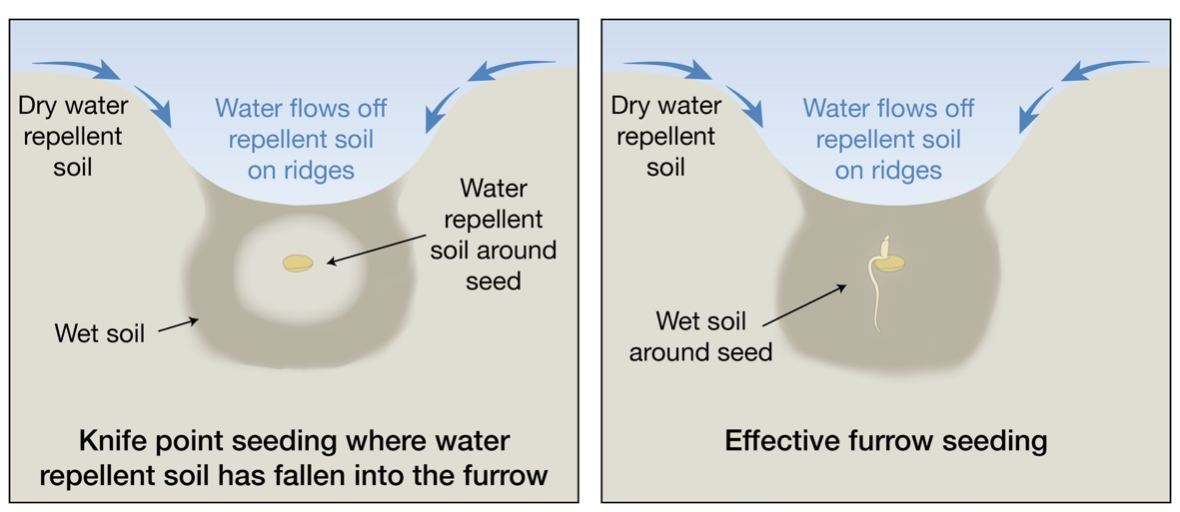
Expression of water repellence may be increased in areas where mechanical working, including dry-seeding, disturbs dry soil. The mechanisms of this are possible associated with the disruption of residual roots, removal of the standing stubble and the breakup of other preferred pathways for water entry. There may also be other mechanisms, such as the disruption of pore connectivity that are as yet either unknown or poorly characterised.
Extension of the cropping area onto soil that was traditionally used for grazing means more crops are being grown on sandy and gravelly soil types which are either prone to water repellence, or have a history of high organic matter inputs that have led to the development of water repellence prior to being cropped.
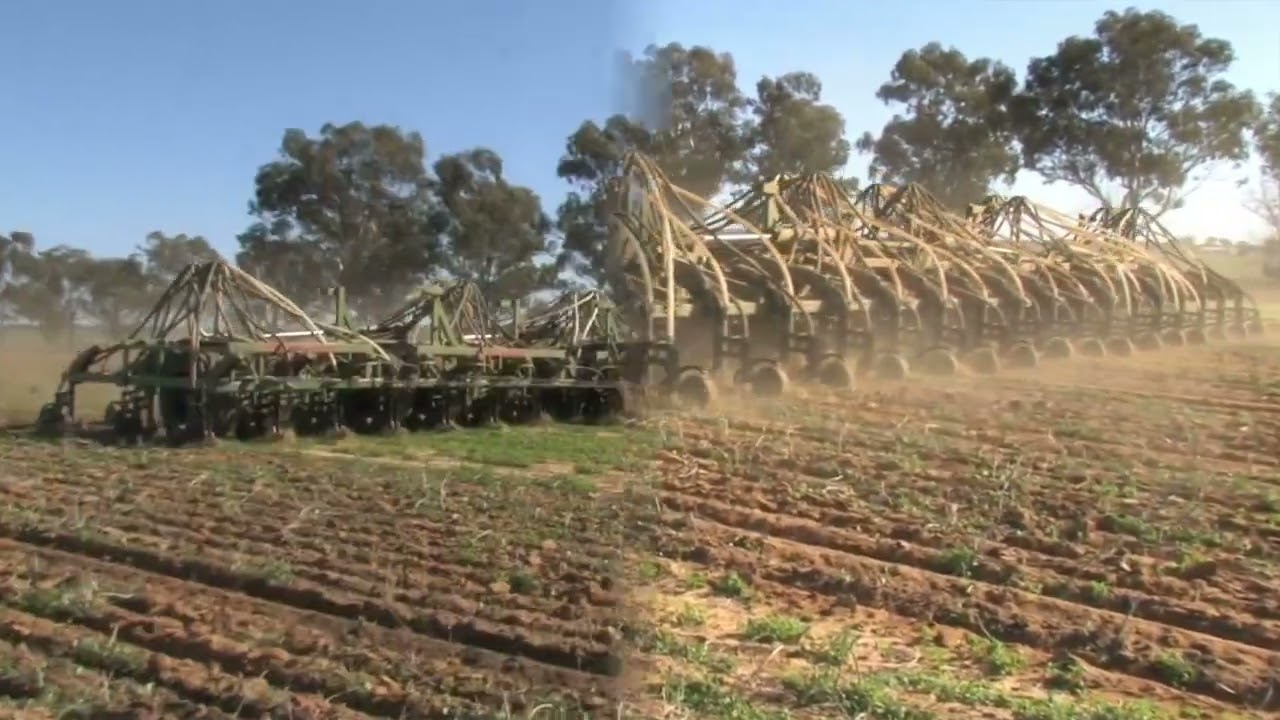
Flow of water repellent topsoil around knife points
Dry-seeding increases the amount of water repellent soil flowing around knife points into the base of the furrow where the seed is placed. From Soil Quality: 7 Soil Water Repellence (Davies et al. 2022). Video footage: DPIRD; voice talent, still images and illustration concept: Stephen Davies, DPIRD; illustration drawing and video editing: Science with Style.
Diagnosing soil water repellence
Assessing severity
Crop symptoms of soil water repellence
Visual indications of water repellence are staggered crop emergence, poor crop establishment (which often presents as large and frequent gaps in the crop row), ponding of water on, or run-off from the soil surface following significant rainfall, and the presence of significant patches of dry soil shortly afterwards. These visual symptoms are not only indicative of soil water repellence but may be related to other causes such as soil compaction, herbicide damage or blockages of machinery.
Where such indicators are related to soil water repellence, an apparent increase in their expression does not necessarily mean that water repellence is getting worse, as it can be related to effects of season (timing and intensity of rainfall and temperature) or to effects of agricultural management.
On-farm testing for water repellence using droplet penetration tests
Assessments of water repellence can be done in the field using readily available materials. A simple and direct measure is the time taken for a water droplet to enter the soil. Alternatively, the concentration of ethanol that is required to penetrate the soil is also a common assessment. The molarity of ethanol (MED) test is provided by some commercial laboratories. On farm, this can also can be determined using beverages with different alcohol concentration or with a diluted industrial grade methylated spirits solution. The greater the molarity of ethanol in water required to penetrate the soil within 10 seconds, the more severe the level of soil water repellence.
Testing can be done by collecting samples of dry topsoil and doing the droplet penetration test in a dish or can also be conducted direct on the soil surface. If taking this approach, it is important to scrape away the surface 5 mm of soil before commencing testing. In some situations, water may infiltrate the top 5 mm of soil but below that, it won’t. Situations where this may occur are caused by:
- Baking of surface soil in the sun can reduce repellence at the surface, however the soil beneath remains repellent.
- In some soils, an armoured layer of heavy sand grains on the surface allows water infiltration, however below these surface grains, water repellence is heavily expressed.
- Organic matter on the surface can absorb water before it reaches the soil.
Simple water droplet penetration test
- Soil must be dry; summer or autumn will give peak repellence measurement.
- Scrape away the top 5 mm of surface soil away to create a flat surface.
- Use an eye dropper or squeeze bottle with a nozzle to carefully place droplets of rainwater or distilled water on soil surface
- Measure the time it takes for the droplets to infiltrate the soil. Use the ratings chart below to determine the level of repellence.
2-molar ethanol droplet penetration test (abbreviated MED)
Diluted industrial grade methylated spirits can be prepared as an in-field modification to the MED test. The concentration of 23.9 ml industrial grade methylated spirits per 200 ml water produces a 2-molar ethanol solution. This method is similar to the water droplet test but uses the diluted methylated spirits in addition to droplets of water.
- Follow the directions for the water droplet penetration test.
- If the droplet takes longer than 10 seconds to penetrate, repeat with the process with the diluted methylated spirits.
- Measure the time it takes for the droplets to infiltrate the soil. Use the ratings chart below to determine the level of repellence.
Scales of repellence
Rating for soil water repellence | Molarity of ethanol test (MED) | Simple water droplet penetration test | 2-molar ethanol droplet penetration test |
---|---|---|---|
Non-repellent | Water infiltrates in dry soil in 10 seconds or less | Water infiltrates in dry soil in 10 seconds or less | |
Low | >0-1.0 | Water takes longer than 10 seconds but less than 60 seconds to infiltrate | Water takes longer than 10 seconds but the 2-molar solution takes less than 10 seconds to infiltrate (repellent) |
Moderate | 1.2-2.2 | Water takes longer than 60 seconds to infiltrate (moderate – severe repellence) | |
Severe | 2.4-3.0 | The 2-molar solution takes more than 10 seconds to infiltrate (strongly repellent) | |
Very severe | >3 |
From Soil Quality: 7 Soil Water Repellence (Davies et al. 2022); Soil Quality: 2 Integrated Soil Management (Pluske et al. 2018); Australian Soil and Land Survey Field Handbook (National Committee on Soil and Terrain 2009).
Further reading
Book Australian soil and land survey field handbook
National Committee on Soil and Terrain (2009). CSIRO Publishing.
Spatial assessment/prediction
The expression of soil water repellence varies across space and time. In space, there is variation not only within a paddock and between paddocks, but between adjacent patches of soil. In time, variability in the expression of soil water repellence occurs within a season and between seasons, but also can be apparent in minutes and hours.
Where the variability in the expression of soil water repellence needs to be assessed, tests of soil, such as outlined in the previous section, will need to be repeated using samples taken from various paddock zones and soil types.
Some of the variability may be at a small scale (e.g. chaff dumps) and simply be recognised as areas to avoid when soil sampling for repellence, while other areas may be of sufficient scale to warrant differential management.
Differences over space and time can be detected more easily using remote sensing techniques, for example Normalised Difference Vegetation Index (NDVI) and visual colour (Red Green Blue, RGB) imagery from satellites and drones. Other methods, including electrical conductivity, x-ray imaging and thermal imaging are currently under investigation. Once validated, such instruments could be deployed using drones, robotics or other vehicles to enable rapid assessment of soil water repellence and the extent of its impacts on crops and pastures.
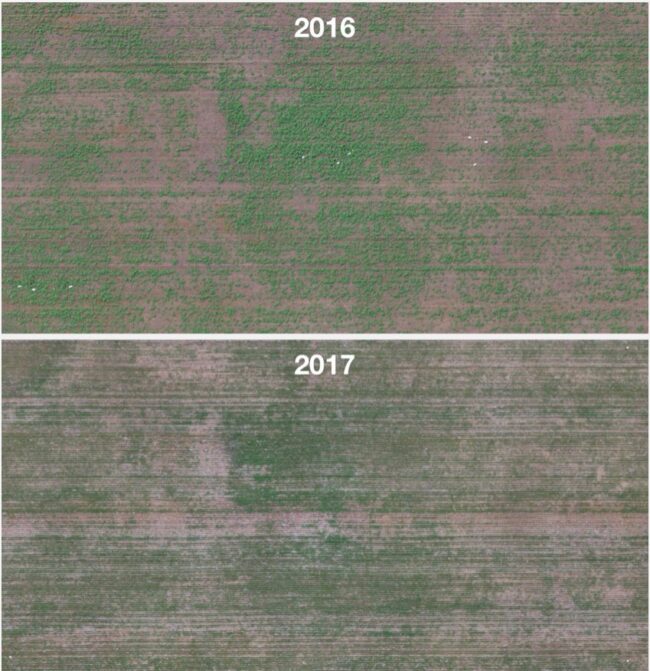
Sources of variability
The sources of variability in water repellence can be numerous and include differences related to the soil, historic and recent inputs of organic compounds and management.
-
Soil mineralogy, in particular clay content, affects the occurrence and severity of soil water repellence. It is unusual for soil with greater than 10% clay content to exhibit water repellence. Both occurrence and severity increase at lower clay contents.
-
Previous vegetation factors, including plant type and biomass, affect the formation and deposition of hydrophobic compounds, as well as the presence of root channels that act as preferred pathways for water infiltration and movement.
-
Landscape features and position, such as hilltops, mid slopes and swales affect movement and concentration of water.
-
Previous tillage (depth, timing, type of implement) and other forms of soil disturbance, such as tracks from machinery or animal movement, affect the distribution and expression of water repellence and also the movement of water into and through soil.
-
Collection and concentration of agricultural plant residues, including wind rows, chaff dumps and chaff lines, impact the concentration of hydrophobic organic compounds.
-
Stubble management, including retention as standing stubble, rolling, chopping or burning, affects the amount of organic matter and its placement; and influences whether an effective conduit remains to help move water more effectively into the soil profile (i.e. via standing stubble).
-
Stock and native animal management, including grazing and animal camps. Sheep camps or confined feeding areas, for example, are known as areas of very high repellence.
-
Surface and subsurface movement of water onto or off parts of the paddock, including ponding, seeps and perched water tables impacts the expression of soil water repellence.
Managing soil water repellence
The strategy for managing water repellence will be influenced by severity, soil types, area of the farm that is affected and presence of other constraints. Take the following steps to develop a water repellence management strategy:
- Diagnose water repellence.
- Determine the soil types affected.
- Assess the occurrence of other constraints.
- Develop a management strategy.
Mitigation
Mitigation options manage soil water repellence to allow crop and pasture production in the repellent soil. Strategies include improving the effectiveness of furrow sowing, stimulating the natural microbial degradation of waxy compounds or delaying sowing until after rain.
The efficacy of furrow sowing can be improved by using:
- winged points or boots
- paired (twin) row seeding boots
- ribbon seeding
- sowing close to the previous year’s crop row
- using banded wetting agents (surfactants) with knife points, especially when dry sowing.
These strategies do not remove or reduce the repellent soil in the long-term, but they are usually cheap to implement and can be applied over a large area of affected soil. The downside is that they generally need to be re-applied every season and they do nothing to address other interacting soil constraints which, if present, will significantly limit yield.
When seeding with knife points, dry repellent topsoil will typically flow around the point and into the seeding row with the seed, exacerbating the effect of repellence. Use of winged points or winged paired-row seeding boots allows seed to be placed on an undisturbed (firm) and often moist seedbed. This, combined with greater grading of repellent soil out of the furrow, can increase plant emergence. Systems that have good separation of seed and fertiliser can also benefit plant establishment in repellent soil with low soil moisture.
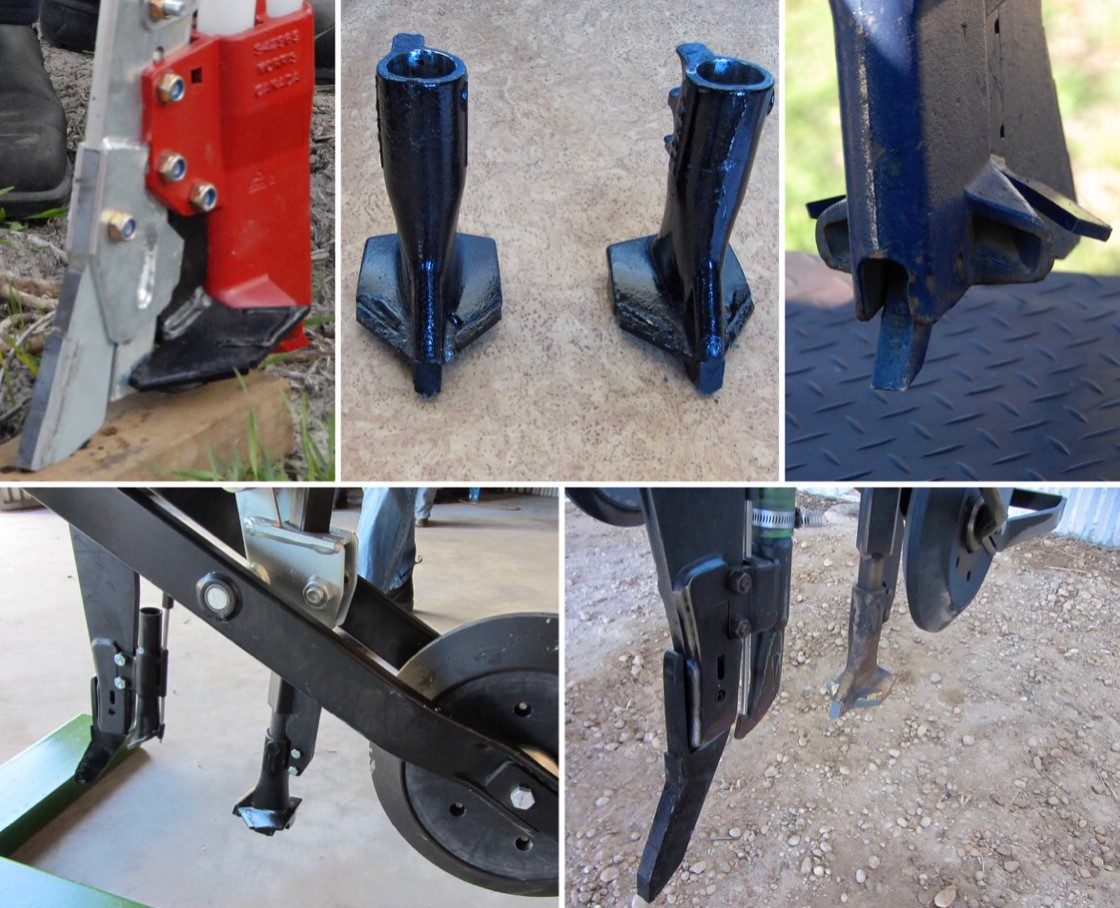
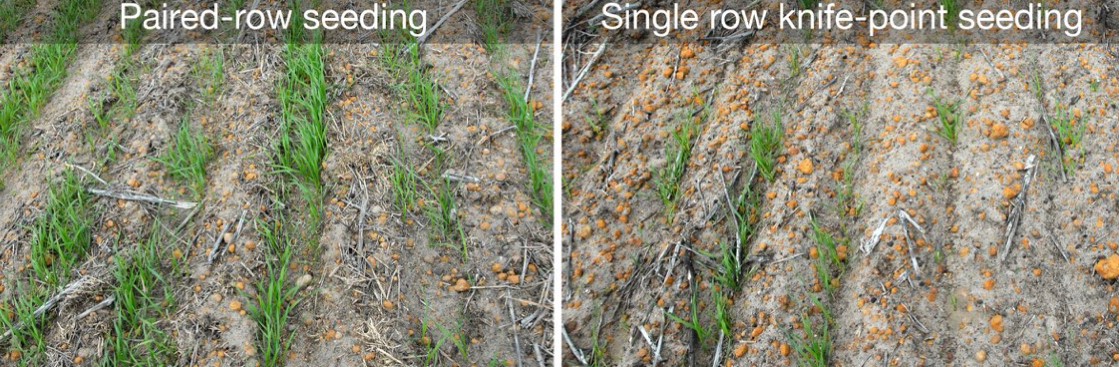
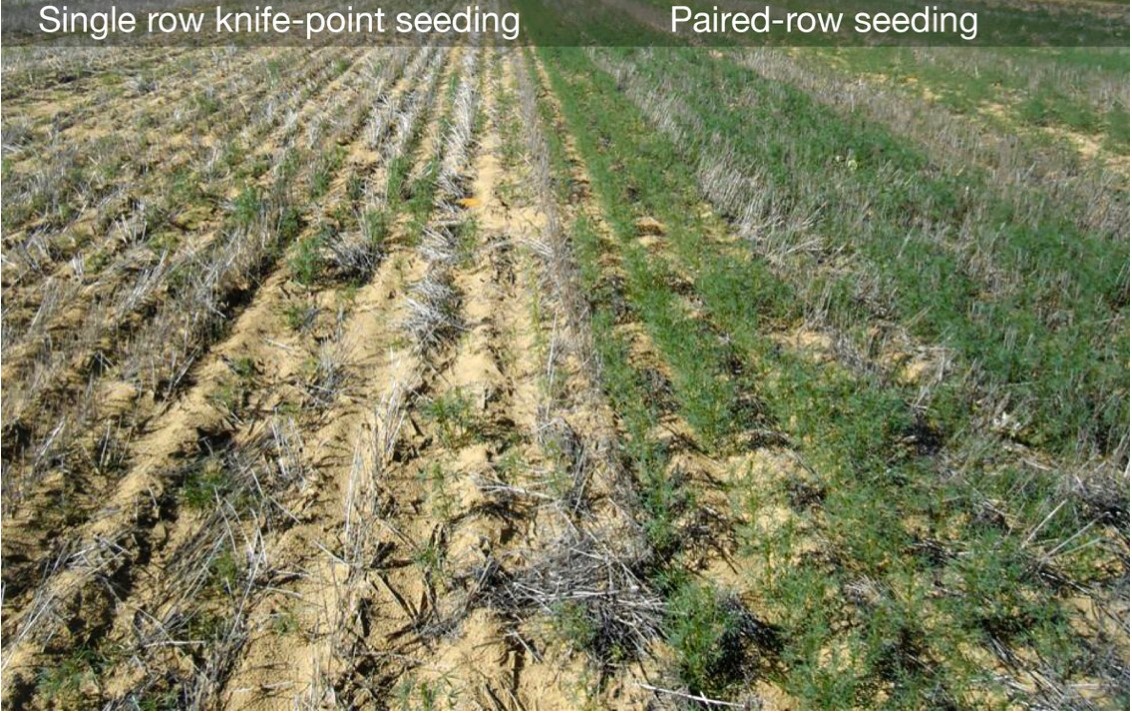
Soil wetting agents (soil wetters) contain surface-active agents (surfactants), which can reduce the surface tension of water at the soil surface and improve water entry into repellent soil. Soil wetters can be banded either on the furrow surface, or in the furrow near the seed. Soil wetters can improve crop establishment, but their impact on yield is variable.
Banded application
On deep sand and sandy gravel soil, the efficacy of banded wetting agents has been inconsistent. This compares to forest gravel soil in the south-west agricultural region of Western Australia, where the benefits are larger and more consistent.
The benefit of soil wetters is greatest under dry conditions at seeding when soil water repellence is expressed more strongly. Use of soil wetters when wet-seeding rarely shows a benefit. Dry conditions in winter also increase the likelihood of achieving a benefit with soil wetters. Research has shown an average yield response ranging from 12-21% when dry-sown compared to -5-3% when wet-sown across a range of repellent soil types.
Yield responses to soil wetters have been more consistent in cereal crops than break crops in field trials, especially in the more responsive forest gravel soil.
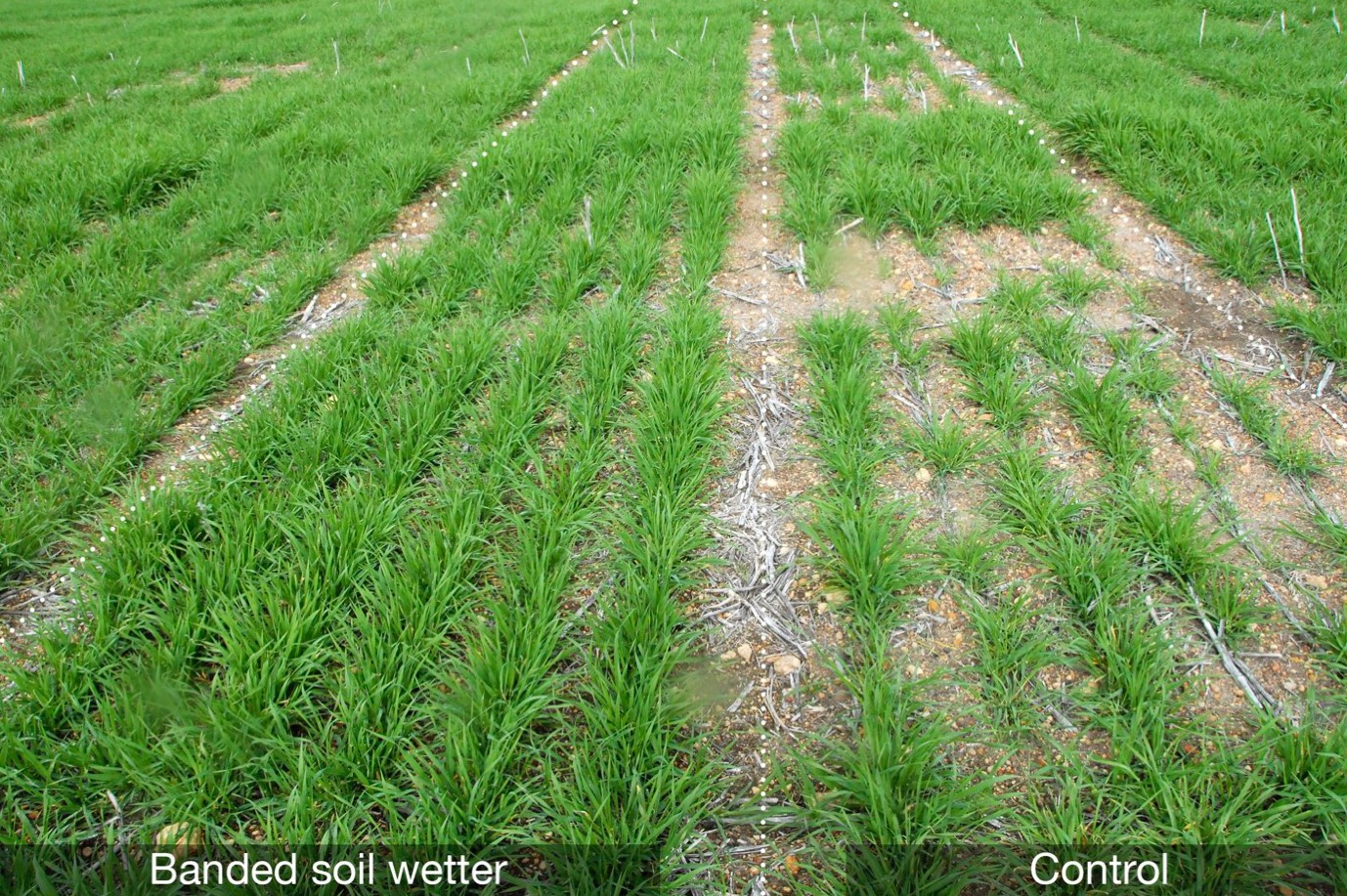
Blanket application
Biannual blanket application of soil wetters is an alternative for repellent, forest gravel soil. However, higher application rates on a per hectare basis can increase the cost compared to targeted, banded application. Yield responses to blanket wetters have been variable but can be used to target most severe and responsive areas within paddocks. The average cereal yield response from blanket wetter on responsive gravel soil is 21% (0.25 t/ha) with 43% of comparisons having a significant positive response in field trials.
Seed coating
Coating seed with soil wetters requires lower rates of product than banding or blanket application. The benefits to crop establishment and growth can be similar to banded soil wetters in responsive situations, but responses have been highly variable and show little benefit in seasons with good germinating rainfall.
No-till usually increases the severity of repellence because organic matter is concentrated in the soil surface layers and the breakdown and turnover of this organic matter is slower compared to situations where the soil and associated organic matter is more disturbed by cultivation. However, no-till preserves remnant root pathways and biopores, down which water can flow and infiltrate repellent surface soil more effectively.
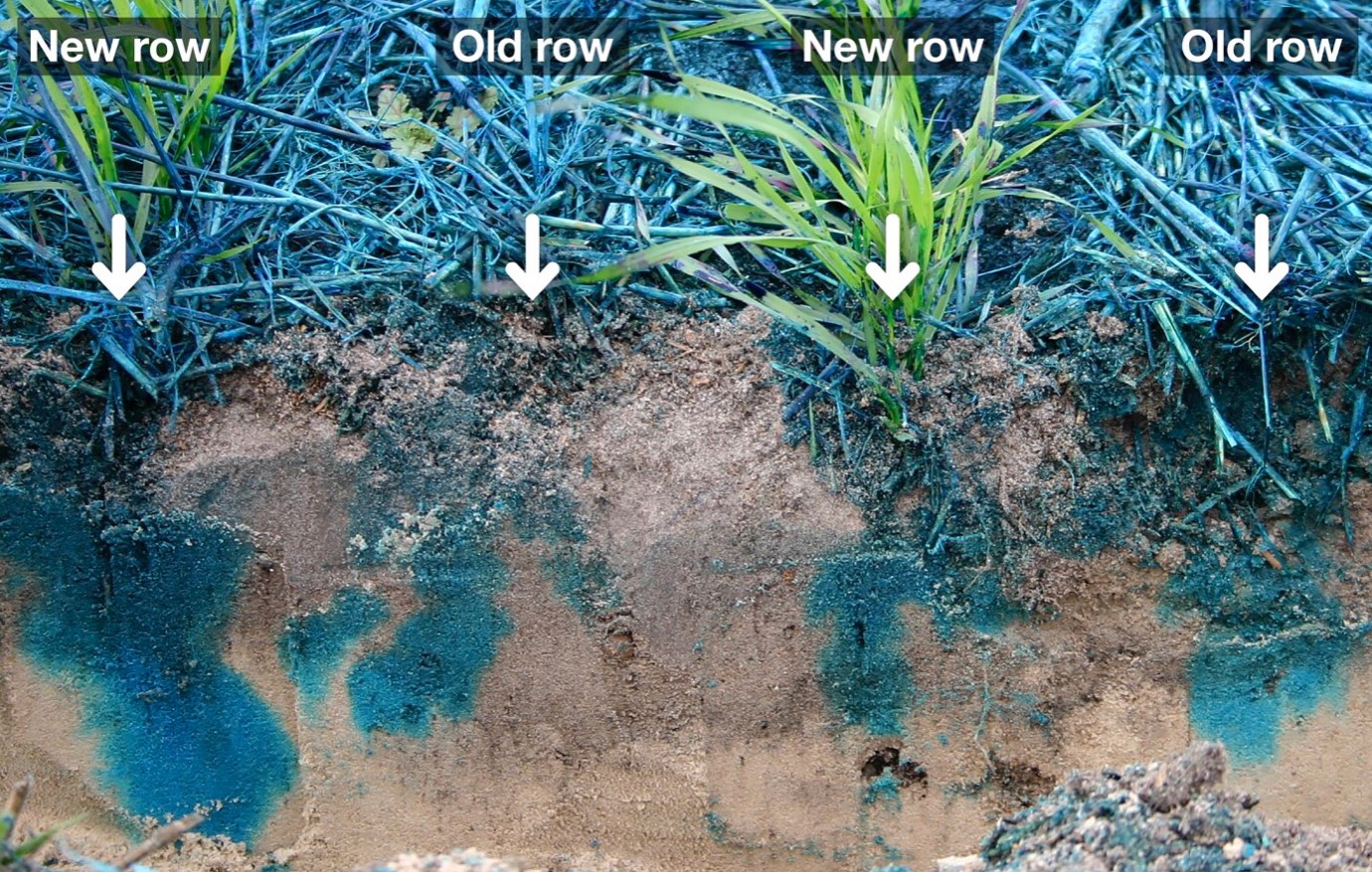
Near-row seeding refers to seeding crops next to or on the previous season’s row. Near-row seeding under no- or minimal-tillage has been shown to increase crop establishment compared with inter-row seeding. Grain yield can also be increased, especially when there are no other major soil constraints. Seeding close to the previous year’s crop rows on a water repellent soil can take advantage of increased water infiltration down intact remnant root pathways benefiting crop performance, particularly in dry years.
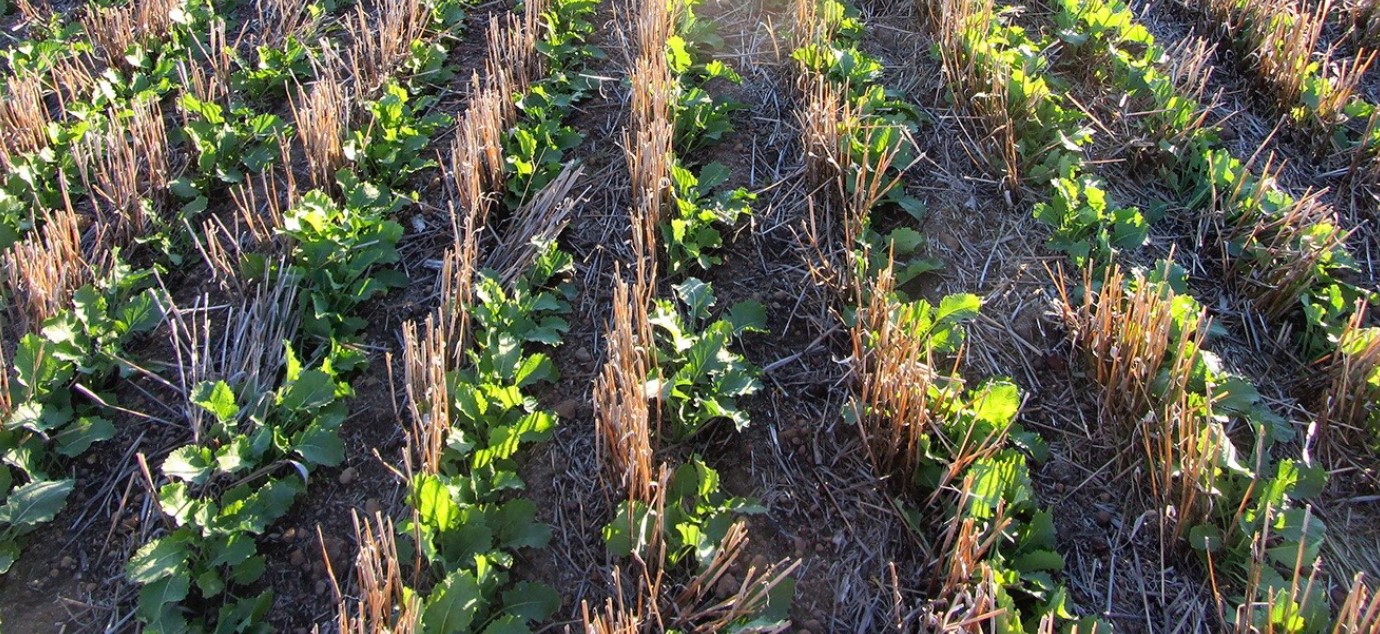
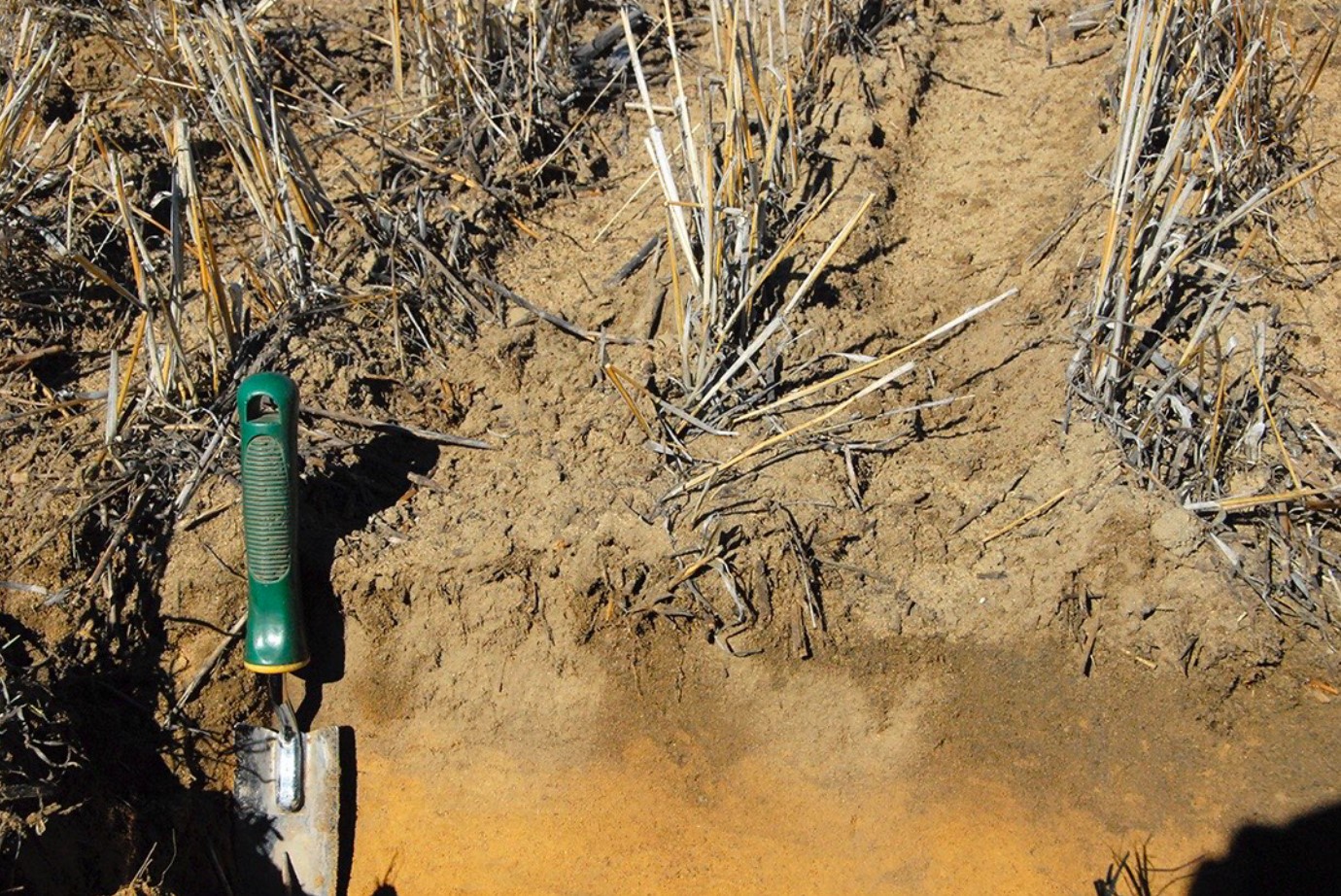
Soil bacteria capable or decomposing organic waxes that cause repellence have been isolated from a range of locations, including water repellent soil in the south-western agricultural region. Inoculation of water repellent soil with these wax-degrading isolates was shown to decrease repellence in controlled environments in the laboratory, but these benefits have not yet been observed in the field. This is most likely due to competition for resources from, and predation by, existing microflora in the soil.
Enhancing the activity of naturally occurring wax-degrading bacteria in soil by providing the most conducive environment for their growth has been much more successful. The addition of lime to acidic water repellent sand can decrease soil water repellence, partly through a ‘claying effect’ of the fine lime particles, and partly through enhancing the environment for the microbial population, thus increasing the numbers of wax-decomposing bacteria and the microbial breakdown of hydrophobic organic compounds. Plant root exudates and decaying residues can supply food and water essential for bacteria, in addition to the physical root structure enabling preferential water flow and providing a pathway for water to enter the soil. Field experiments have shown that communities of wax degrading bacteria can be up to 10 times grater in the root zone (rhizosphere) that in the inter-row. This provides support for near-row seeding as a tool for managing water repellent soil.
Expression of repellence can be particularly severe in autumn, when soil is still dry but humidity is increasing. Delaying seeding on water repellent soil until after significant break of season rainfall can improve seed germination, crop establishment, and in some cases grain yield. It is therefore recommended to avoid dry-seeding of paddocks with repellent soil, leaving them until the end of the seeding program, where possible. Weeds are typically problematic on repellent soil associated with staggered germination – particularly on dry-seeded paddocks, and delaying seeding until after opening rains can provide an opportunity for better weed control prior to seeding.
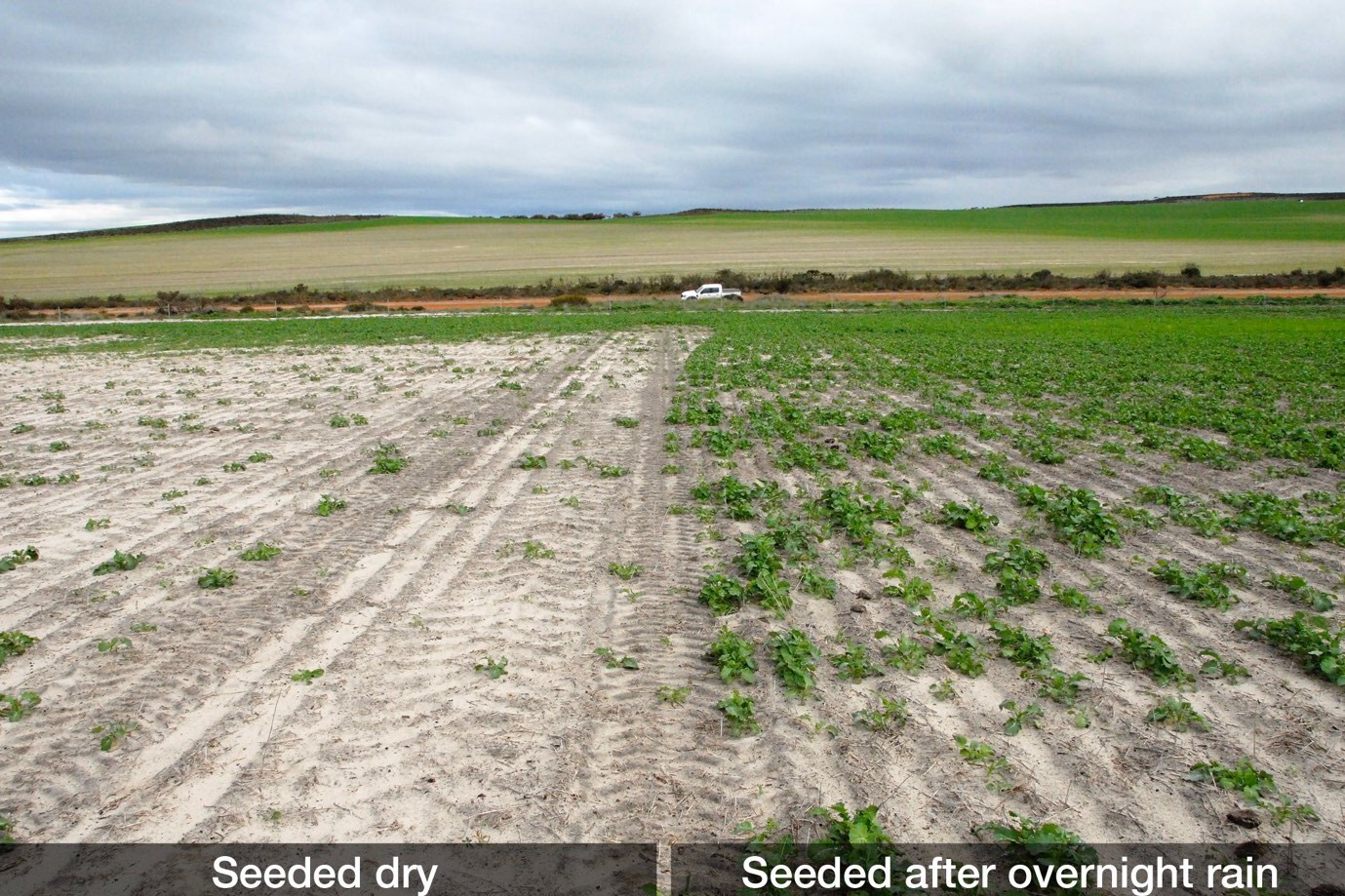
Avoiding dry-seeding is an option in situations where mitigation or amelioration is not possible, for example, due to topography, budget, or available machinery constraints. Where dry sowing is unavoidable, near-row seeding can mitigate the impact of water repellence on germination.
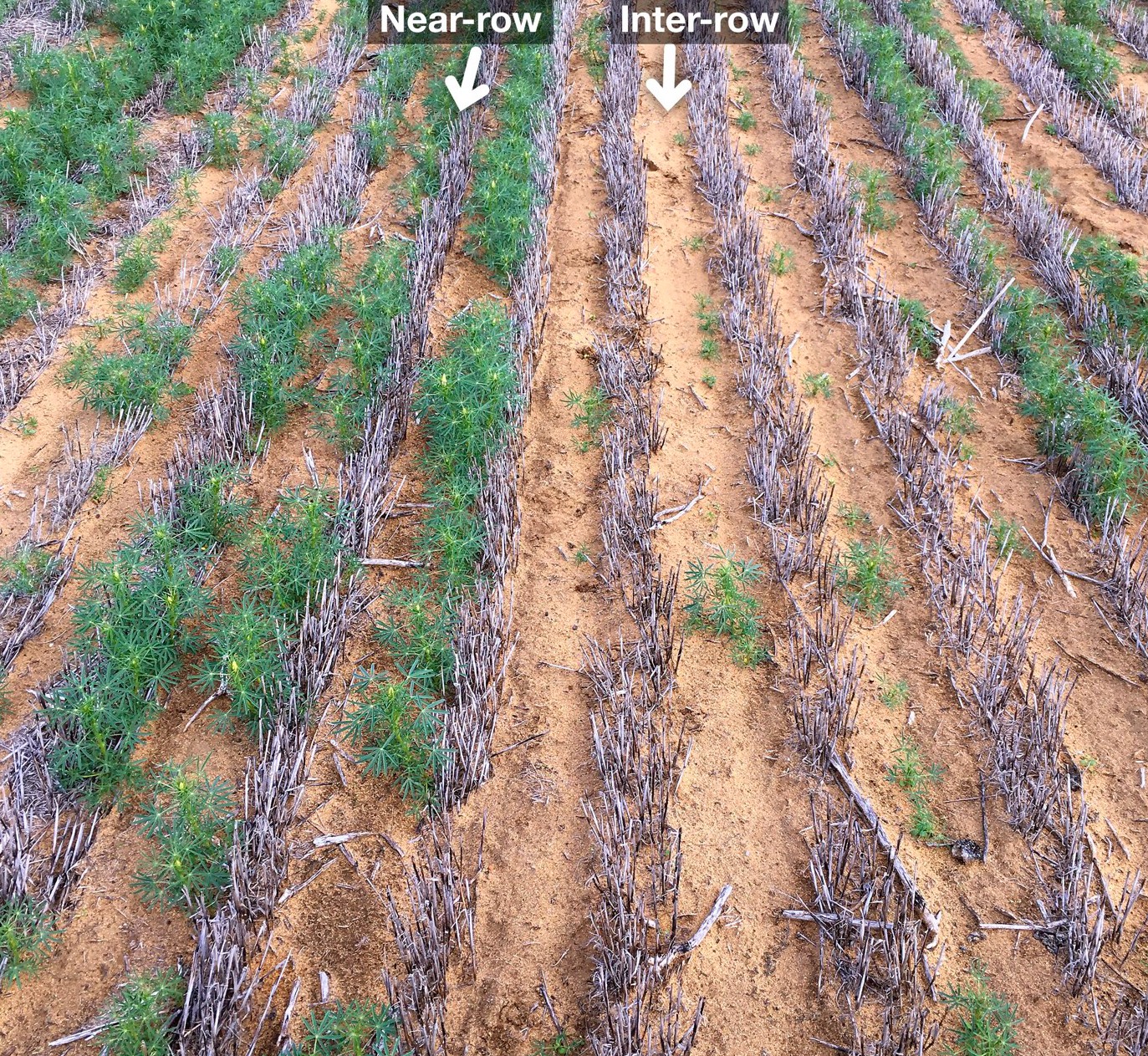
Blackwell P, Davies S, Best B (2013). Evaluating approaches to improve furrow sowing on water repellent sands. West Midlands Group, Research Annual, 2012 season. [online]
Blackwell P, Hagan J, Davies S, Bakker D, Hall D, Roper M, Ward P, Mathews A (2014). Smart no-till furrow sowing to optimise whole farm profit. Paper presented at GRDC Grains Research Updates, Perth. [online]
Cook A, Richter I (2017). Crop establishment on non-wetting sand. Eyre Peninsula Farming Systems, Summary 2017. [online]
Davies S, Betti G, Harte L (2016). Long-term assessment of management options for water repellent sandy gravel soils. West Midlands Group, Research Annual, 2015 season. [online]
Davies S, McDonald G, Betti G, Harte L, Poulish G, Bakker D, Devlin R, Jenkinson R (2016). Assessing options for managing water repellent gravels. Paper presented at GRDC Grains Research Updates, Perth. [online]
Desbiolles J, McBeath T, Barr J, Fraser M, Macdonald L, Wilhelm N, Llewellyn R (2019). Seeder-based approaches to mitigate the effects of sandy-soil constraints. In: Proceedings of the 2019 Agronomy Australia Conference, 25 – 29 August 2019, Wagga Wagga, Australia. Australian Society of Agronomy. [online]
Kerr R, Ward P, Roper M, Micin S, Davies S (2019). Managing water repellency with minimal soil disturbance. Paper presented at GRDC Grains Research Updates, Perth. [online]
McBeath T, Desbiolles J, Gupta V, McKay A, Llewellyn R, Davoren B, Moodie M, Correll R (2016). Sowing strategies to improve the productivity of crops in low rainfall sandy soils. Paper presented at GRDC Grains Research Updates, Adelaide. [online]
McBeath T, Llewellyn R, Gupta V, Davoren B, Shoobridge W, Kroker S (2017). On-row sowing for brome competition on non-wetting sands. Mallee Sustainable Farming report booklet [online]
McBeath T, Macdonald L, Trengrove S, Moodie M, Desbiolles J, Fraser M, Kookana R, Llewellyn R (2018). Improving crop productivity on sandy soils. Paper presented at GRDC Grains Research Updates, Walpeup. [online]
Roper MM (2005). Managing soils to enhance the potential for bioremediation of water repellency. Australian Journal of Soil Research 43(7) 803-810. https://doi.org/10.1071/SR05061
Roper MM, Ward PR, Betti G, Davies SL, Wilhelm N, Kerr R, Micin SF, Blacker T (2021). Seeding next to previous year’s crop row (near-row sowing) can increase grain yields on water repellent soils. Soil Research 60(4), 360–372. https://doi.org/10.1071/SR21142
Amelioration
Amelioration falls into two broad groupings; strategic deep tillage to bury and/or dilute the repellent topsoil, and the addition of clay-rich subsoil either through spreading or delving on duplex soil. These techniques tend to be expensive but can have substantial and long-lasting impacts on productivity. These strategies have significant economic and environmental risks if not implemented correctly. For this reason, amelioration should be targeted at areas that are likely to have large yield responses once the repellence constraint is overcome. Highly repellent soil that has reasonable water and nutrient holding capacity is likely to be a good option for amelioration.
Soil amelioration often has the potential to overcome a number of constraints which can increase the size and longevity of the benefits.
Complete soil inversion uses a mouldboard plough, square plough, or modified one-way disc plough to bury repellent topsoil as a distinct layer in the subsurface soil, replacing it with wettable subsurface soil that has been brought to the surface. When carried out effectively, soil inversion almost completely eliminates topsoil water repellence, although, in cases of incomplete or poor inversion, soil retains some repellence. It is expected that the decrease in surface water repellence from successful inversion will last for at least ten years and the repellence of the buried topsoil typically declines to a low level over the first few seasons following inversion.
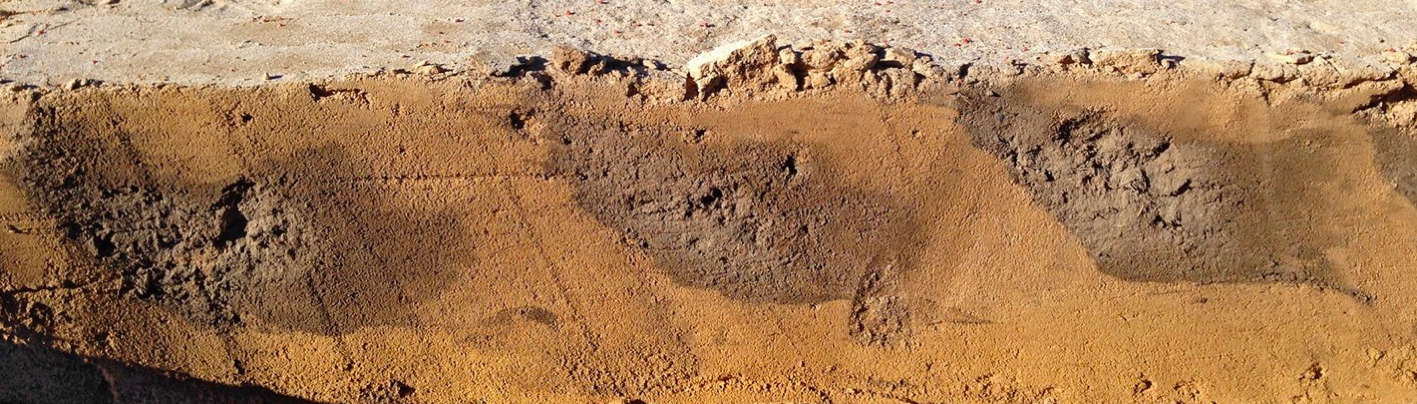
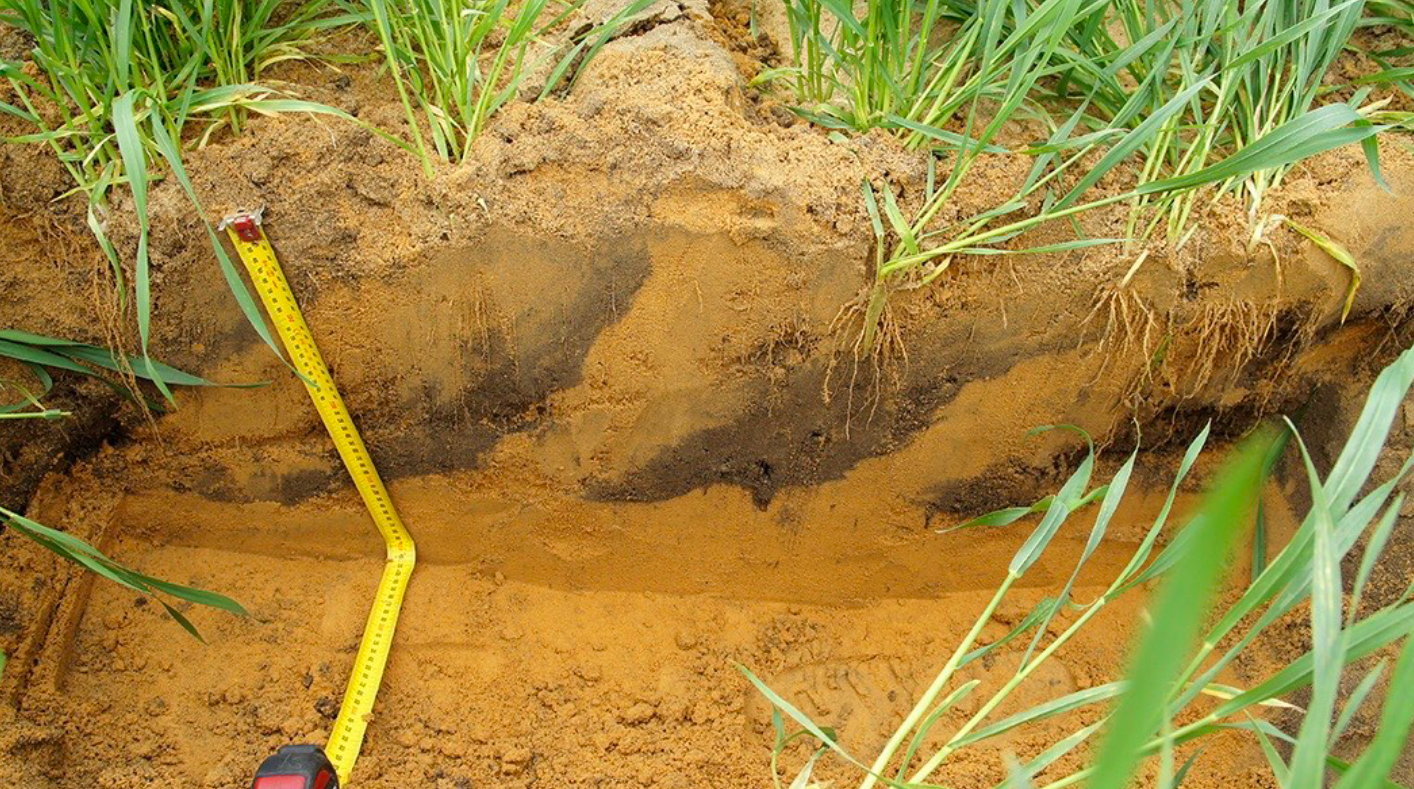
Mouldboard ploughs are designed to invert soils so in moist soil with correct setup they will bury a higher proportion of the topsoil than other inversion ploughs. There are many aspects of a mouldboard plough that can be altered to achieve the most effective inversion possible (see Setting up mouldboard ploughs to optimise soil inversion video).
One-way disc ploughs can be modified as a relatively low-cost implement to invert soils. Every second disc is removed to allow space for the soil to turn over. Larger discs are fitted, up to 750 mm diameter, to engage the subsoil and bury repellent topsoil. The discs often have scalloped edges to improve rotation and ability to cut through roots. Breakout on each disc is increased, hydraulically if available. Some ploughs have a heavy main beam but others may require addition of weight on the end of the plough to help it stay in the ground when deep ploughing. Plough setup needs to be optimised for soil type and soil moisture conditions. Modified one-way ploughs are often used on repellent gravel soils.
Benefits
Crop grain yield responses to soil inversion can be large and sustained for ten or more years. In field trials, soil inversion produced average increases in cereal grain yield of 30–60% (0.54–0.88 t/ha) in the first two years, depending on soil type. Responses tend to be lower on low fertility deep sand, and higher on deep sandy duplex and repellent gravel soil.
Typically, the reliability of positive yield responses in the first few years after inversion ploughing is high, with the exception of inversion ploughing on deep sand where only 56% of sites responded positively (2009–2018). In all cases, the non-responsive comparisons of inversion on deep sand were a result of either drier seasons, or poor crop establishment as a consequence of herbicide damage, wind damage or surface crusting when coupled with dry conditions. Soil with higher yield potential typically maintained benefits to cereal yield for a longer time than soil with lower yield potential (e.g. pale sand). Benefits on pale deep sand, whilst high in the first few years, declined significantly thereafter, except for severely repellent sites. For sandy duplex and gravel soil, average cereal crop yield benefits in excess of 0.5 tonnes per hectare were maintained for three or more years after amelioration, with significantly positive responses in 88% of cases for sandy duplex soil and 75% for gravel soil.
The yield response of canola to soil inversion is quite reliable (significant positive benefit in 67–100% cases, depending on soil type). The capacity for canola growth to compensate for poorer crop establishment was an advantage on inverted soil. For lupin, plant establishment on severely repellent soil was typically greatly improved by inversion – with average yield responses that were particularly evident on pale sand.
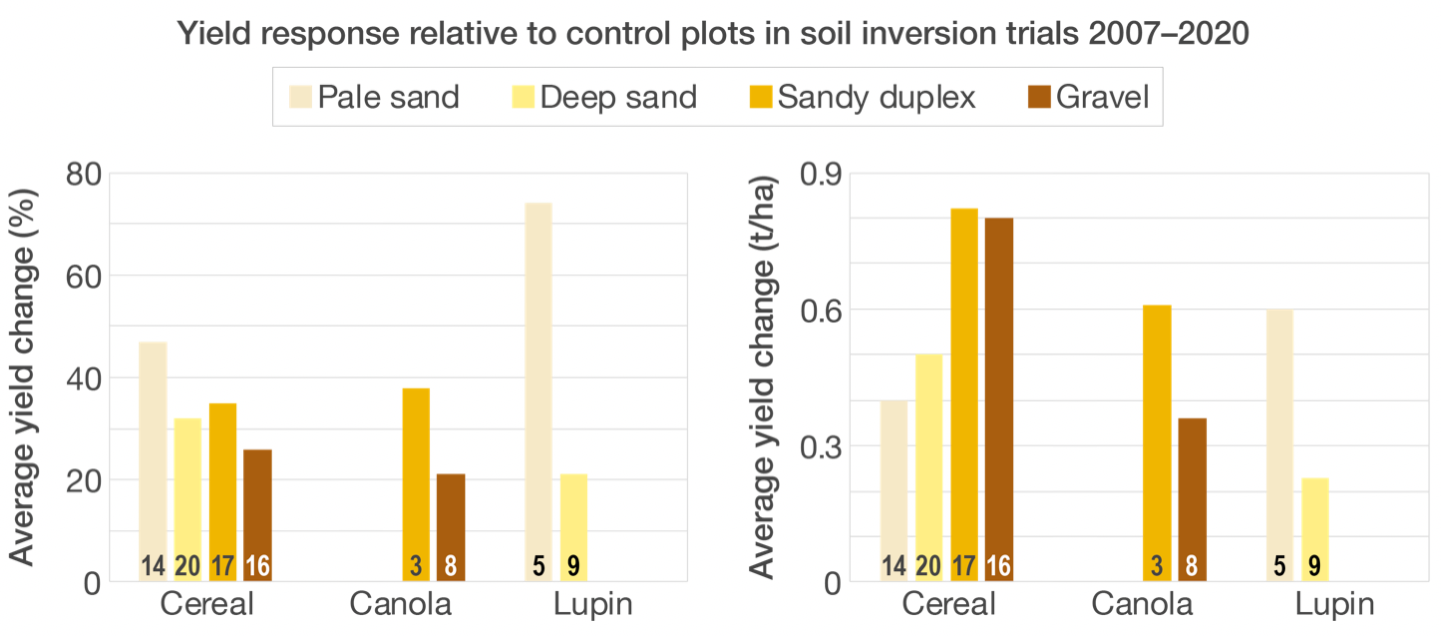
On average the grain yield response to mouldboard and deep working one-way disc ploughs are similar. Use of either inversion tool can be readily justified depending on grower needs, finances and soil types. The cost to purchase and to modify a one-way plough is much lower compared to a mouldboard plough – and the differences in performance are relatively small, particularly when compared to soil that has not been ameliorated. Other agronomic benefits and risks can also influence the decision. Mouldboard ploughs provide more complete inversion and burial of the weed seed bank and the reduction in soil water repellence can be greater. One-way disc ploughs are cheaper to purchase and can more effectively ride over rocks and stumps. In some cases, the mouldboard plough can bring up excessive subsoil clay and cause surface sealing and crop establishment can be more difficult.
Other benefits from soil inversion may include amelioration of subsurface soil compaction, incorporation of lime into the soil profile to manage soil acidity, burial of weed seeds, lower risk of stubble-borne disease, enhanced water-holding and potential nutrient-holding in the subsurface soil.
Risks
Strategic deep tillage will leave soil in a loose and soft condition. This can make seeding and seeding depth control difficult as seeding bars will tend to sink and equipment will leave deep wheel tracks. As a result, germination and establishment of crops can be negatively impacted – particularly in the first season after amelioration. Packing and firming the topsoil using rock rollers, rubber-tyred rollers or other packers can help, but it can be difficult to achieve the right balance between packing to establish good seed bed conditions and re-compacting the soil. In some cases where controlled traffic is employed, wheel tracks can be left undisturbed giving a firm foundation for trafficking of machinery. Purpose-built, light and simple seeding bars may also be used for seeding ameliorated soil. These typically do not have deep working points so seed cannot be sown too deep. Alternatively, some growers have had success with broadcast spreading of seed and pressing it in with packers, while others have developed or purchased equipment allowing them to plough and seed in the one pass. Cereal cultivars capable of emerging from depth are recommended in the first few years.
The reduced concentration of organic matter at the soil surface after tillage means that pre-emergent herbicides have increased activity, which may result in considerable crop damage and even seedling death. Rates of application need to be adjusted to allow for this, or even foregoing the use of pre-emergent herbicides for the first few seasons after amelioration
Another risk of soil inversion is the redistribution of subsurface soil which has chemical constraints to the surface, adversely impacting plant growth. Most commonly, this is in the form of acidic subsurface soil, or soil with sufficient clay content that it forms a ‘crust’ on the new soil surface and subsequently decreases crop emergence. It is critical to carry out chemical analysis of subsurface soil to at least the depth of disturbance prior to carrying out inversion to help identify any potential risks. For example, if highly acidic subsurface soil is brought to the surface, aluminium toxicity can inhibit plant establishment and lime will need to be applied to the soil surface, and preferably mixed, as well as growing more acid-tolerant crops immediately following inversion.
Deep soil mixing is carried out using implements such as deep working off-set disc ploughs or rotary spaders. Rotary spaders have a horizontal shaft which rotates, typically at around 90 revolutions per minute in the direction of travel. Sets of curved tines, typically triangular-shaped spades are attached to the shaft that both bury topsoil on the downward stroke and lift subsoil on the upward stroke resulting in deep soil mixing. Rotary spading is effective at burying some topsoil and lifting seams of subsurface soil to the surface. In repellent soil, these seams act as preferred pathways for water entry, decreasing topsoil water repellence. However, due to the mixing action of the spader, some repellence remains. This is most often evident in soil with severe water repellence, and low clay and silt contents throughout the spading depth. Deep ripping prior to spading, allowing a deeper working depth, can improve the outcome. Forward operation speed significantly impacts mixing effectiveness. Rotor rotation speed remains consistent regardless of forward speed so at faster speeds the ‘bite length’ between each spade increases and uniformity of mixing declines, especially at depths below 200 millimetres. At slower speeds, topsoil is mixed more uniformly to the working depth. There is, however, limited evidence from field experiments that operating the spader at speeds below that recommended by the manufacturer improved productivity or agronomic outcomes. The benefit from rotary spading can last 4–10 years.
Deep working offset discs can also partially deep mix soils. The largest discs with a diameter over 1000 millimetres can cut to 300–350 millimetres depth. The first set of discs on the machine throw the soil one-direction then the next set throw the soil back again, at the same time mixing and blending the soils. Penetration of the offset discs into hard soil can be difficult; deep ripping prior to using offset discs can help ensure full depth working of the discs and more effective mixing.
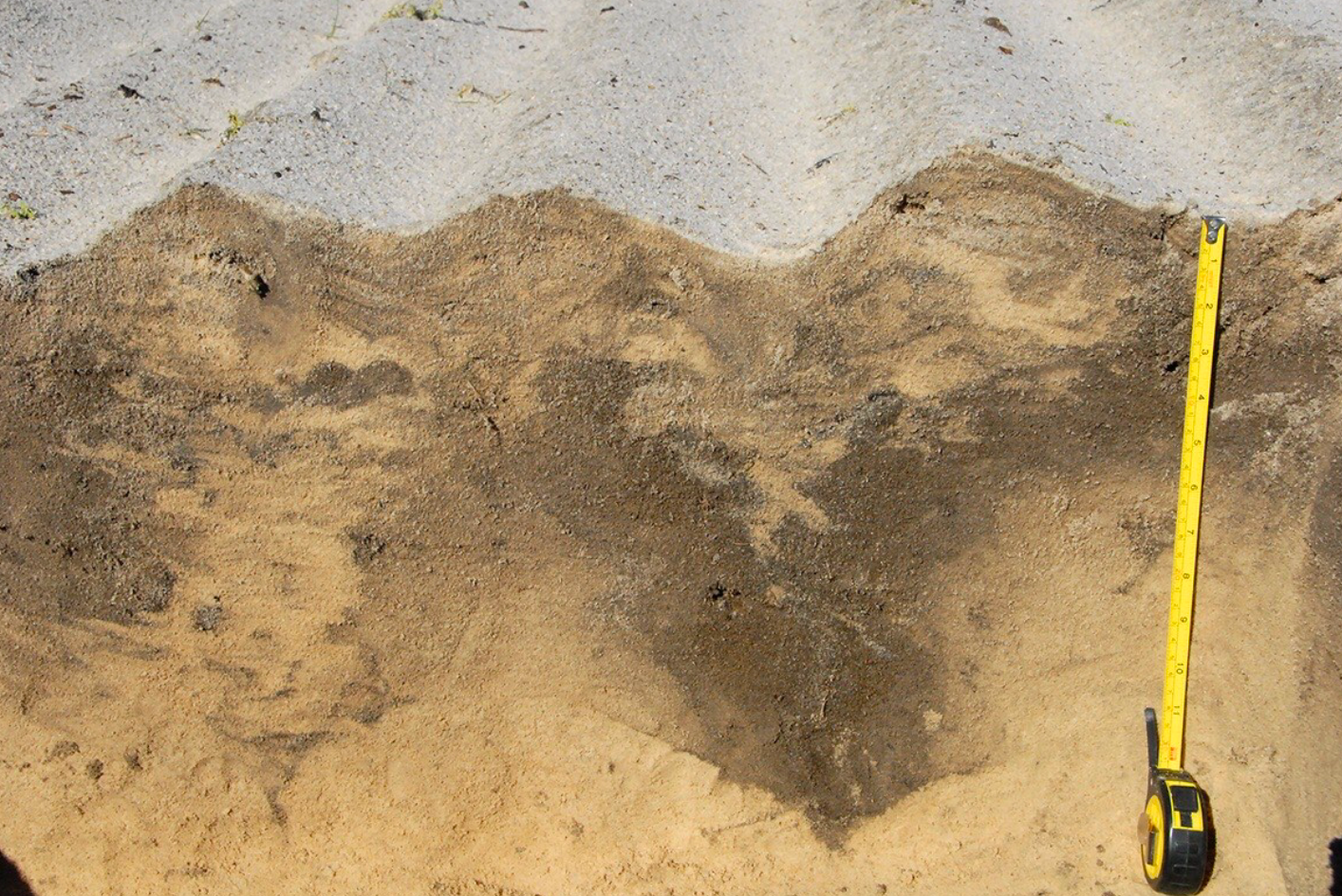
Benefits
Cereal grain yield responses using rotary spaders are of a similar magnitude to those from inversion. In field trials, the rotary spader had more consistent positive yield responses in the first two years on deep sand, with 84% of comparisons giving significant increases. Over all trial years, the proportion of sites with a significant positive response in cereal crop yield to soil mixing was 75%, 79% and 93% for pale sand, deep sand and sandy duplex soil respectively. Negative or nil yield responses of lupin to spading, resulted either from sandblasting of the lupin crop resulting in seedling mortality, or dry seasons with periods of high drought stress – especially during early growth. Canola responses were strongly related to soil type with greater response on sandy duplex.
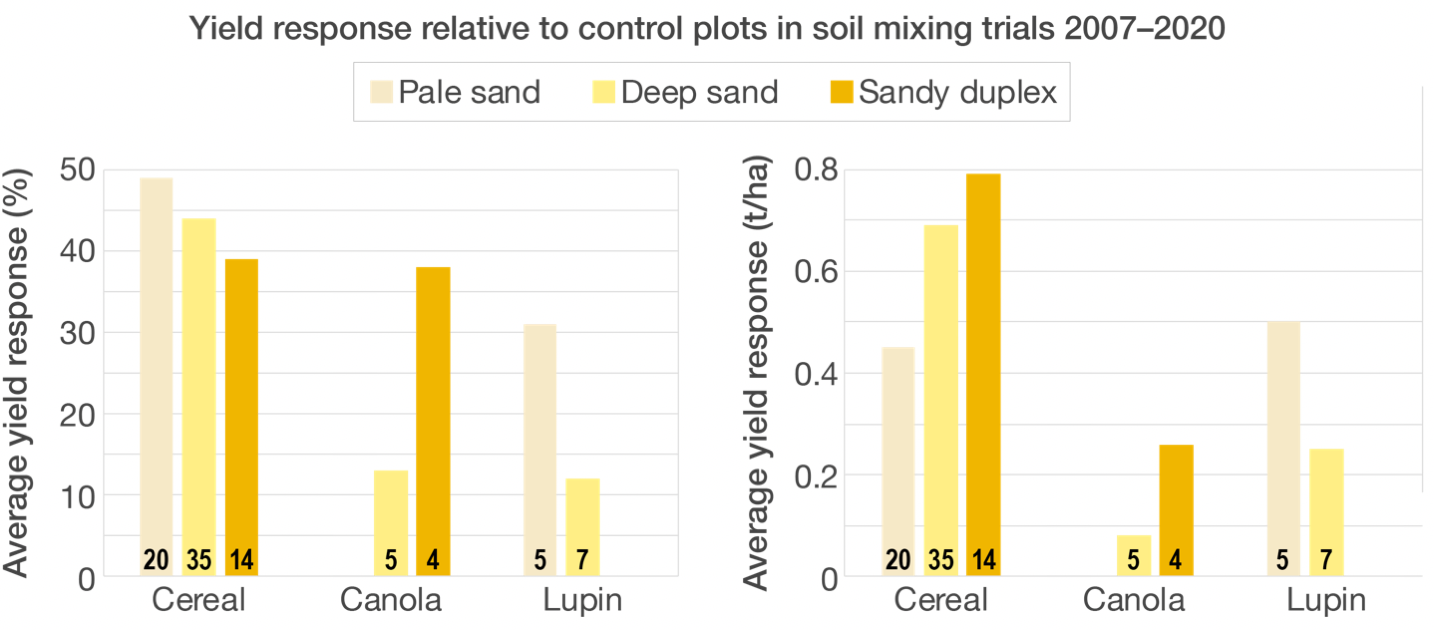
Other benefits from deep soil mixing may include less subsurface soil compaction, incorporation of lime deeper into the soil profile, burial of weed seeds leading to some additional weed control, and the incorporation and mixing of nutrients into the subsurface soil which with improved root interception, and soil remaining wetter for longer, may increase nutrient use.
Risks
Deep soil mixing results in an extreme risk of wind erosion. Implementing the practices when the soil is wet and establishing a cereal cover crop as soon as possible is the principal way to minimise the risk. Deep mixing at times of the year when winds are less strong and less frequent can also reduce the risk of erosion.
Clay spreading involves the spreading and incorporation of clay rich subsurface soil into water repellent sand. The increased surface area of the clay particles and the coating of the organic matter can help to overcome water repellence, with the benefits known to last for decades.
Clay is often sourced on-farm and generally, involves excavation of a clay-rich subsurface soil from a large pit which is then spread over the repellent sand. Spreading is typically undertaken using carry graders (requires clay to be further distributed over the surface with a smudge bar), purpose-built broadcast spreaders or heavy-duty multi-spreaders. Clay-rich subsurface soil is typically spread at rates of 100–450 tonnes per hectare, aiming to increase the topsoil clay content to 3–6% clay which is required to overcome repellence. Once spread, a range of deep cultivation approaches (e.g. tines, off-set discs, harrows, rotary hoe) are used to incorporate the clay into the top 100–200 millimetres. Increasingly, rotary spaders have also become a common implement for incorporating clay following spreading or delving (spading also reduces repellence; see Deep soil mixing).
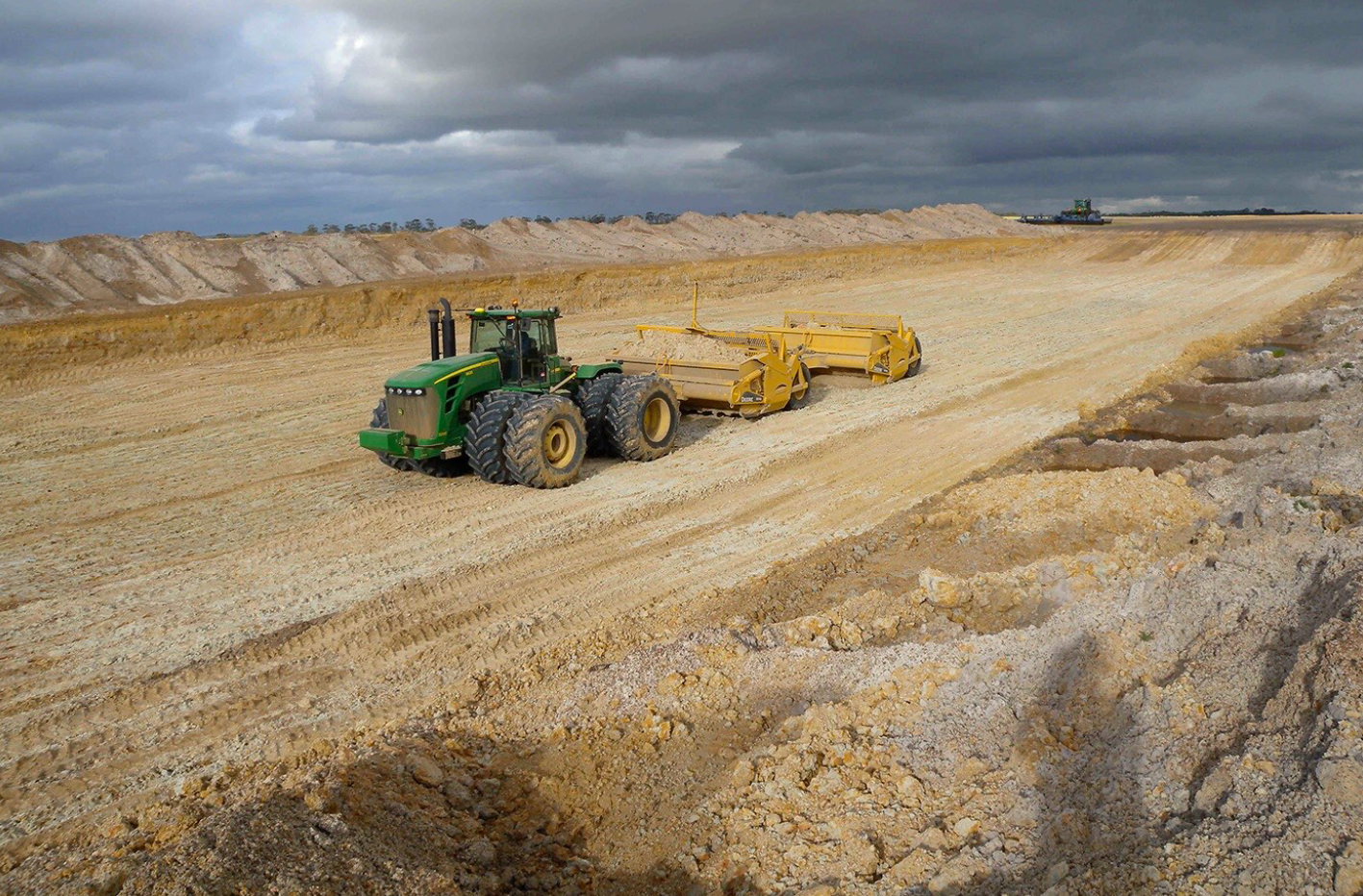
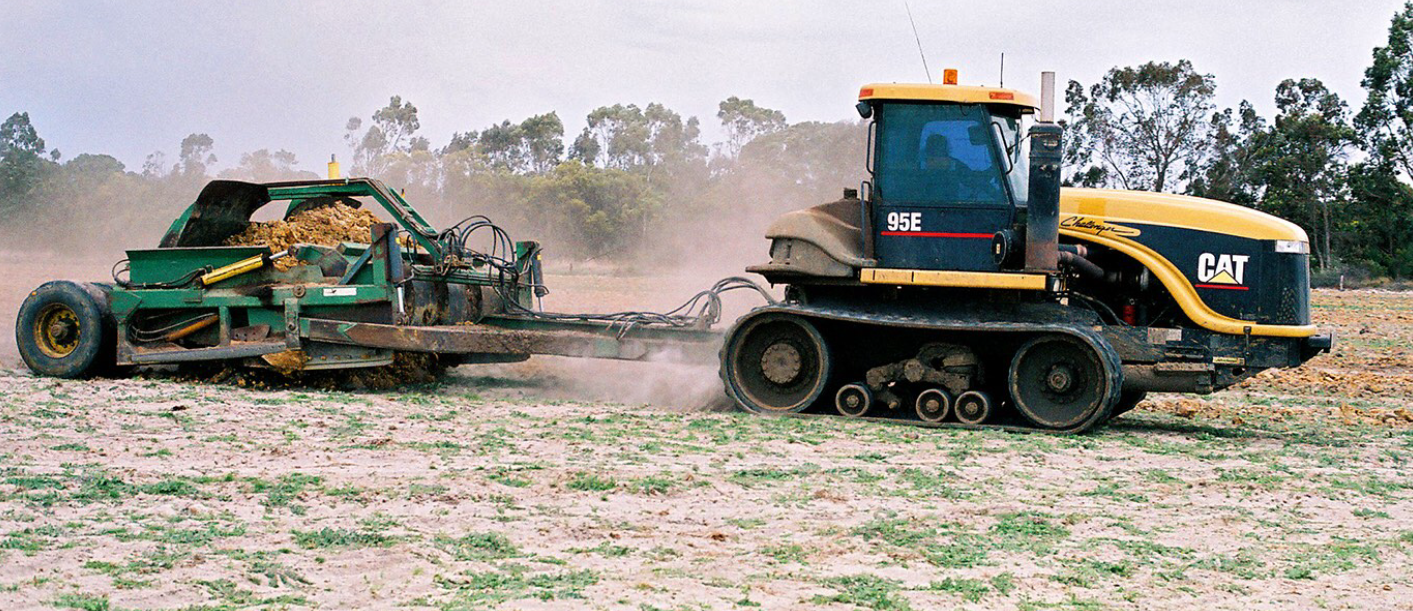
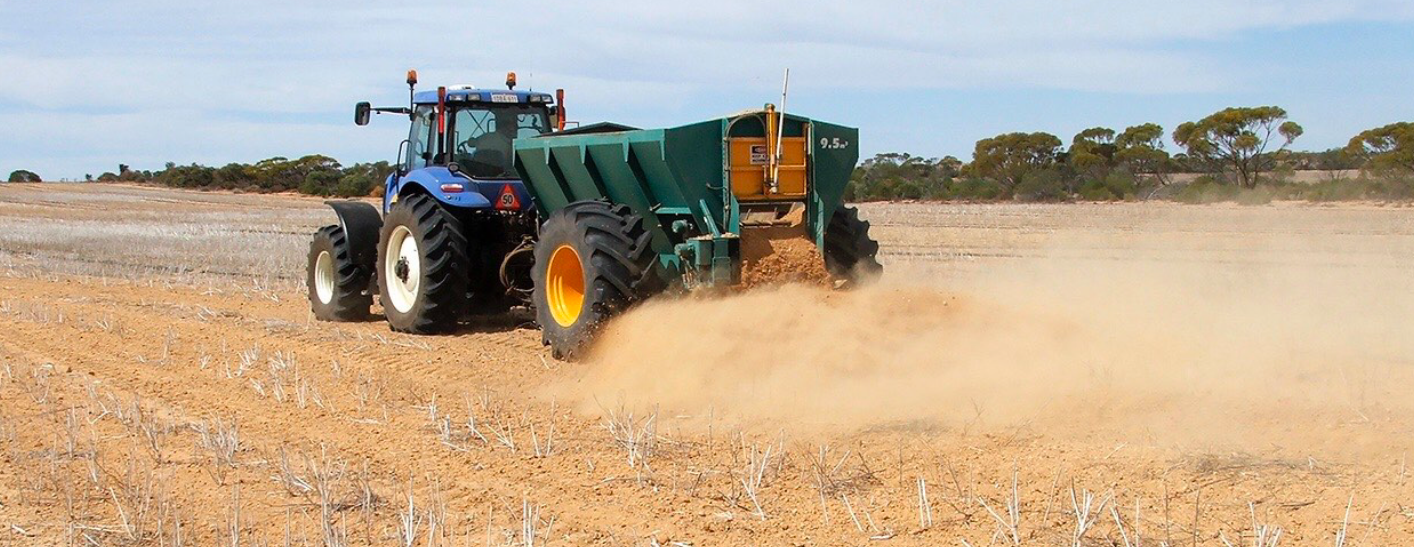
Benefits
In Western Australia, trial results have indicated that clay spreading using multi-spreaders at more moderate rates (100–250 t/ha) can consistently improve crop yield over many seasons. Average increases of 0.3–0.4 tonnes per hectare were observed for cereal grain yield (37–42% greater than where no clay was spread) (n = 6 sites).
Although gains in yield are observed with clay spreading followed by spading, the high cost of implementation means it can take many years to break even. The benefits of clay spreading appear to accrue over the longer term, with minimal benefit in the first three to four years, but more benefit subsequently. Clay addition comes at a high cost but provides a near-permanent amelioration of soil water repellence.
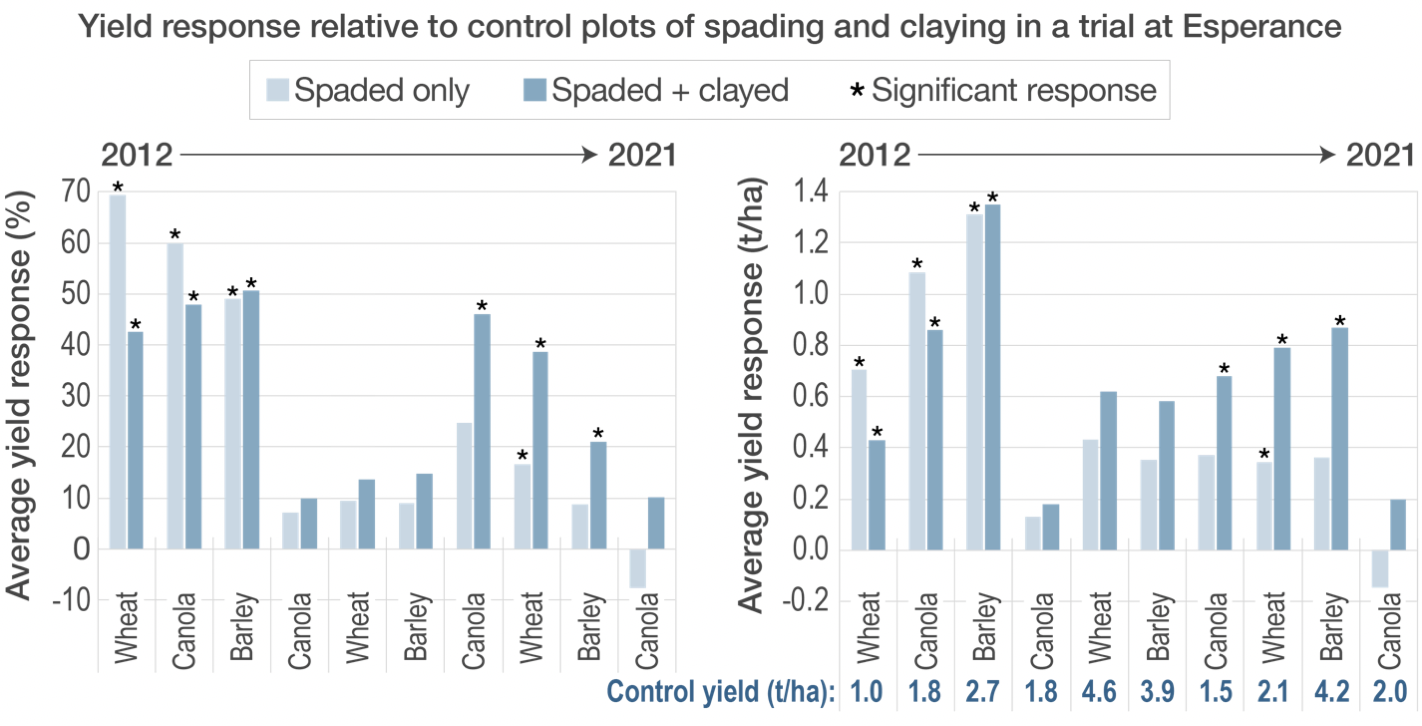
Incorporation of clay subsurface soil can also modify soil pH, nutritional status, moisture dynamics, carbon sequestration, soil stability and biological activity. Improved plant nutrition, particularly potassium from the applied clay, and greater water infiltration are key factors behind improved productivity on clayed soil. Substantial benefits to soil organic carbon stocks (up to 22 t/ha increases, 0–300 mm) have also been associated with increased clay content at several sites, with likely benefits to biological fertility and nutrient retention.
Risks
Claying carried out incorrectly can cause yield decline. Poor incorporation of high rates of clay-rich subsurface soil are a particular concern. Rates of 200 tonnes per hectare or more can be difficult to incorporate effectively and can lead to negative impacts such as soil sealing, poor emergence and restricted root development. Implements for deeper incorporation, such as rotary spaders and large-diameter deep working offset discs help minimise this risk.
High rates of clay applied via a carry grader and inadequate incorporation coupled with dry seasons, poor finishes or mid-season dry spells can result in increasing haying off of crops and decreased grain yield on clayed soil, particularly in lower rainfall parts of the central and northern wheatbelt. An alternative approach to spreading clay, is to spread lower rates using a heavy-duty multi-spreader. This can result in more-even spreading and negates the need for additional smudging to spread heavy ‘windrows’ of clay. At more moderate rates incorporation with offsets and cultivators is often sufficient, reducing the need for more costly deep incorporation required when heavy rates are applied.
Assessing and testing subsurface soil prior to spreading (or delving) is important to avoid using clay that brings with it other constraints to plant establishment and growth. Clay that remains in clumps will not mix with the repellent topsoil and so takes longer to become effective. Clay with deficiencies or toxicities, such as those that are strongly alkaline or acidic, or where toxic levels of boron, salts, carbonates and bicarbonates are present may cause problems for plant growth and production. The rate of application is also a determinant of potential constraints. Moderate to high rates of alkalinity for example, can induce deficiencies in micronutrients, such as manganese deficiency in lupin. However, if low to moderate rates are applied concentrations may not be high enough to be a problem for more tolerant cereal crops, particularly once the clay is diluted through the sandy topsoil. In some situations, rainfall received over time can leach salt and boron, reducing the problem.
Mechanical compaction of subsoil induced by loaded carry graders can also reduce crop yield. Deep ripping after claying can treat compaction and adoption of a controlled traffic farming system can limit the area of re-compaction.
Clay delving uses deep working tines (600 mm or deeper) to penetrate the clay in the subsurface soil layer of texture contrast or duplex soil, while at the same time breaking up compacted or cemented layers above. The tines are fixed, often angled at around 45° so as to lift the clay towards the surface. Following delving, the soil is typically worked with offset discs or a rotary spader to mix and incorporate the clay. If subsurface soil clay is less than 300 millimetres from the soil surface, a spader will introduce more clay from the subsoil as well as incorporating the clay from the surface.
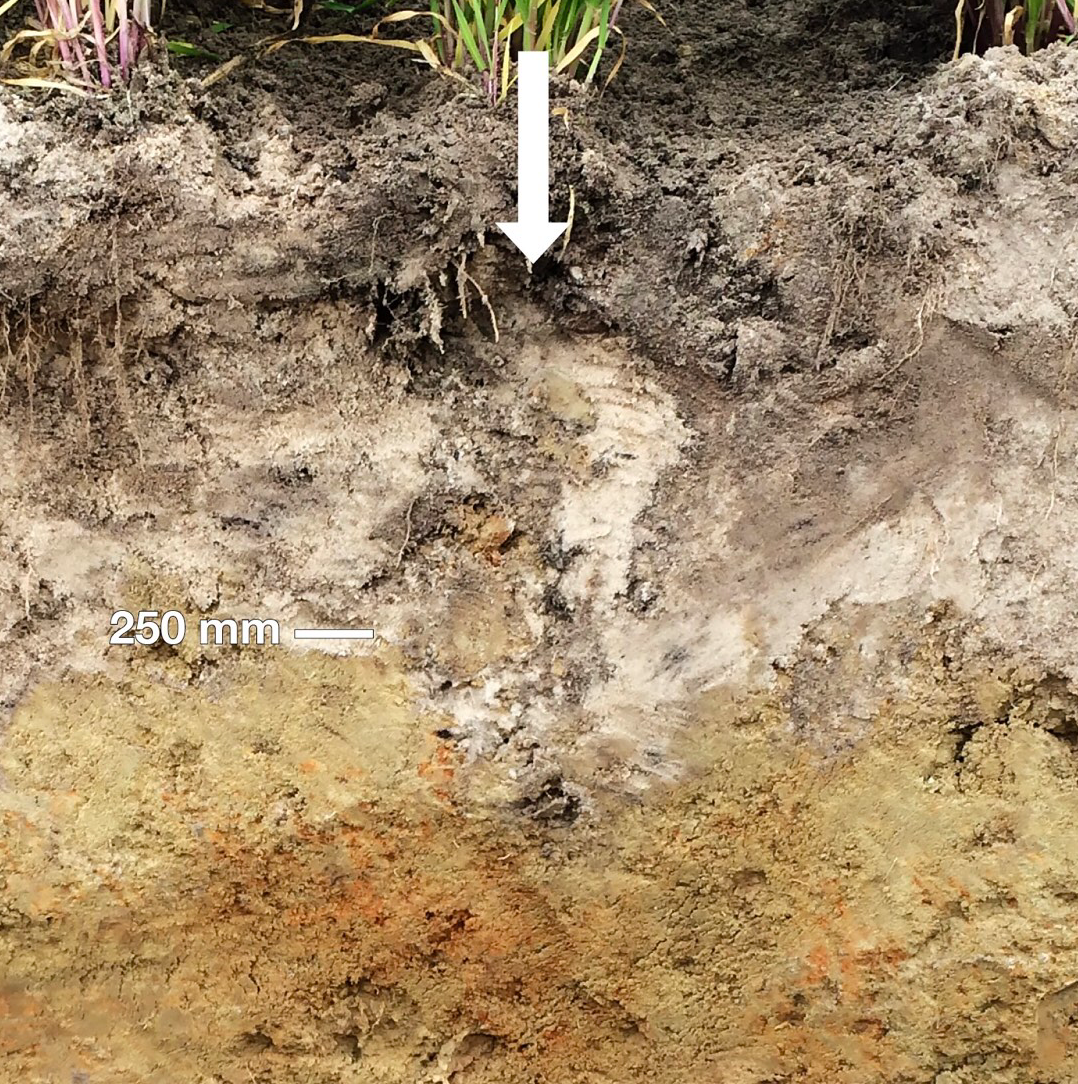
Benefits
Clay delving is generally cheaper than clay spreading so is the preferred approach if the subsurface soil in the paddock contains suitable clay within 600 millimetres of the surface. Delving has the added benefit of distributing clay-rich subsurface soil through the soil profile, rather than mixing it from the surface.
Risks
As the depth to clay-rich subsurface soil will vary across a paddock, the amount of clay brought to the surface will also vary. It is therefore difficult to determine the exact rates of clay being applied when delving. Test strips will help to gauge the rates that will be brought to the surface, but only provide an indication.
Delving must be carried out in the same direction as intended paddock operations as it is extremely difficult to work at an angle to the delve lines. Rolling and/or working along controlled traffic lines can help to manage distinct delve lines across the paddock which reduce trafficability and may result in uneven seeding depth.
(Other Benefits and Risks see Clay spreading).
Deep ripping, also known as subsoiling, involves the loosening of dense subsurface soil typically to a depth of between 300–600 millimetres to improve soil structure, porosity and water infiltration. It is predominantly of benefit for overcoming subsurface soil compaction and has limited application for the management of soil water repellence.
Narrow-tined rippers typically incorporates only 5–10% of the topsoil into soil layers to a maximum depth of 150 millimetres. This ‘mixing’ is passive with topsoil falling into temporary voids around and behind the ripping tines as they pass through the soil.
Deep ripping with topsoil inclusion plates, also known as topsoil slotting, increases the amount of soil mixing and can bury some water repellent topsoil. An opener is added behind the ripping tine that operates just below the topsoil, holding the subsurface soil open to allow loosened topsoil and surface applied amendments to fall into slots. Topsoil slotting allows the deep placement of organic matter or ameliorants.
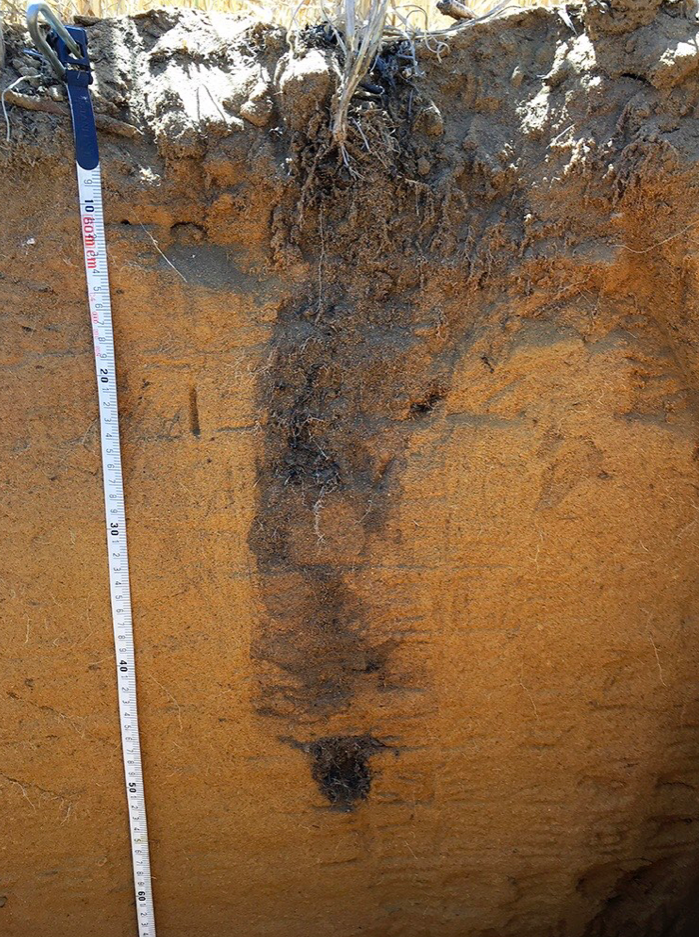
Deep ripping with delving plates (which is not the same as true clay delving) allows subsoil to be lifted in seams to the soil surface, typically from a depth of 150–250 millimetres. Delving plates on rippers provide a continuous angled surface from the back of the ripping-tine point to the soil surface allowing subsurface soil to be lifted into the repellent topsoil.
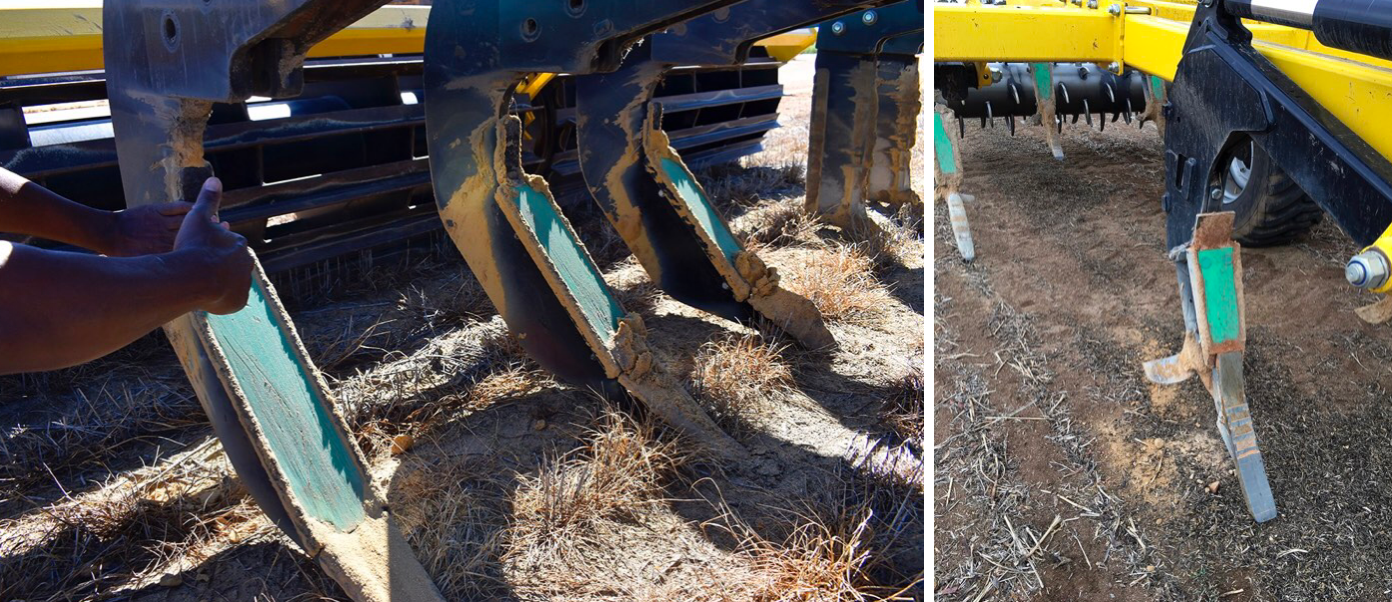
Benefits
Deep ripping to ameliorate soil water repellence alone is generally not cost-effective. It may be beneficial where soil compaction is also a constraint and/or if carried out in conjunction with other amelioration options to address multiple soil constraints that may be present, such as acidity, nutrient deficiency/toxicity, or sodicity. Ripping with delving plates can reduce soil water repellence.
Risks
Risks associated with deep ripping include disturbance of dry soil leading to an increase in the expression of water repellence, bringing excessive clay or hostile subsurface soil to the surface on heavier-textured soil, and the loss of soil structure, all of which can result in poor establishment of crops. Deep ripping (>300 mm) will leave soil in a loose and soft condition within the ripping profile, but is not as extensive as more vigorous methods (See Soil inversion for discussion). Enhanced early vegetative growth of the crop associated with greater water use, can lead to insufficient moisture left in the soil profile to meet crop demand during grain filling and pinched grain in seasons with a dry finish.
Soil loosened by very deep tillage or ripping is prone to re-compaction. Controlled traffic farming systems will reduce this risk and on deep sand, deep ripped areas can remain loosened following amelioration for 4–5 years.
Determining which strategic deep tillage option, or options, best suits your circumstances depends on a number of factors. Of primary consideration should be the soil type and both the presence and type of soil constraints. Secondary considerations include biotic and/or environmental constraints limiting either implementation or response. Finally, factors specific to the individual farming operation and business, such as finances, labour, practicality, etc. that can impact decision making.
Amelioration of soil water repellence can result in large yield benefits, particularly where multiple soil and agronomic constraints, such as compaction, acidity, crop establishment and access to nutrients are addressed simultaneously.
Summary of factors and benefits associated with responsive situations for a range of amelioration options for managing water repellent cropping soil in the south-western agricultural region of Western Australia
Davies S, Hagan J, Reynolds C, Smee V, Lefroy W, Andrew J, Newman P (2015). Long-term responses to soil amelioration – benefits will last more than 10 years! Paper presented at GRDC Grains Research Updates, Perth. [online]
Davies S, Betti G, Edwards T, McDonald G, Hall D, Anderson G, Scanlan C, Reynolds C, Walker J, Poulish G, Ward P, Krishnamurthy P, Micin S, Kerr R, Roper M, Boyes T (2019). Ten years of managing water repellent soils research in Western Australia – a review of current progress and future opportunities. Paper presented at GRDC Grains Research Updates, Perth. [online]
Saunders C, Ucgul M, Godwin RJ (2021). Discrete element method (DEM) simulation to improve performance of a mouldboard skimmer. Soil and Tillage Research 205: 104764. https://doi.org/10.1016/j.still.2020.104764
Schapel A, Marschner P, Churchman J (2019). Influence of clay clod size and number for organic carbon distribution in sandy soil with clay addition. Geoderma 335: 123-132. https://doi.org/10.1016/j.geoderma.2018.08.009
Scanlan CA, Davies SL (2019). Soil mixing and redistribution by strategic deep tillage in a sandy soil. Soil and Tillage Research 185, 139-145. https://doi.org/10.1016/j.still.2018.09.008
Ucgul M, Saunders C, Desbiolles JMA, Davies SL, Parker W (2019). Improving the effectiveness of soil amelioration by optimising soil machine interaction. Paper presented at GRDC Grains Research Updates, Perth. [online]
Ucgul M, Saunders C, Li P, Lee S-H, Desbiolles JMA (2018) Analyzing the mixing performance of a rotary spader using digital image processing and discrete element modelling (DEM). Computers and Electronics in Agriculture 151: 1-10. https://doi.org/10.1016/j.compag.2018.05.028
Avoidance options
Subtropical perennial grass pastures may provide a management option on poor quality water-repellent sand. For example, kikuyu grass (Pennisetum clandestinum) has become well established in the cooler south coast region, and Rhodes (Chloris gayana) – panic (Panicum spp.) grass mixtures are common in the north. Biomass production from subtropical species over summer compared with temperate species increases the potential for production from grazing systems.
Other options include fodder shrubs, farm forestry or return to native vegetation.
Integrated management
Multiple soil, biological and climate constraints impact cropping systems in Western Australia. Strategies to manage soil water repellence have benefits in the management of other constraints. This may be enhanced by the use of more than one strategy, for example strategic deep tillage (e.g. deep ripping as well as soil mixing/inversion) as well as incorporating amendments such as fertilisers, clay, lime and/or organic matter.
Strategic deep tillage together with the addition of ameliorants may be used to address interacting constraints, to stabilise or improve soil structure, ameliorate subsurface acidity, or to improve subsoil fertility. A one-off soil inversion using a mouldboard plough can have the dual benefit of reducing soil water repellence as well as helping to manage weed seedbank and herbicide resistance. Similarly, burying crop stubble can help to reduce stubble-borne diseases and may reduce the risk of frost.
Soil inversion and deep soil mixing to alleviate soil water repellence can have the added benefit of reducing subsurface soil compaction. Deep ripping is also used to break up compaction layers and can provide preferential pathways for water entry. Soil management may include the use of more than one strategic deep tillage method to optimise management of multiple constraints. Deep ripping to ameliorate soil water repellence alone is generally not cost-effective, but returns can be increased where soil compaction is also a constraint.
Similarly, clay delving to ameliorate water repellence as a deep ripping effect that can further benefit crop yield. Up to an additional one tonne per hectare of wheat yield has been attributed to the deep ripping effect of clay delving; part of which is associated with the decrease in water repellence where the clay increases surface area and lessons the surface tension allowing water entry.
Approaches to ameliorate soil water repellence provide opportunities to assist with the management of soil acidity. Deep soil cultivation to ameliorate soil water repellence provides an opportunity to apply soil amendments, such as lime, into the soil profile. Spreading lime in advance to soil inversion (e.g. mouldboard ploughing) will help to incorporate the lime to depth to address subsurface soil acidity. Lime needs to be placed 250 mm or deeper in the soil profile for this to occur.
Rotary spading can effectively incorporate large volumes of spread lime to depths of 300-400 mm in a single pass, thereby ameliorating subsurface soil pH. Spading is expensive, typically costing between $120-150 per hectare, a large additional cost on top of liming but, in many cases, it provides significant yield increases and may shorten the time it takes to ameliorate an acidic soil by 5-10 years, thereby providing a more rapid payback.
Deep ripping will also allow some incorporation of lime at depth, but mixing is limited and injecting the lime behind ripping tines will lead to large bands of lime with limited capacity to react with surrounding acidic soil.
Application of clay by spreading or delving may increase the pH of the surface soil, provided that the subsurface clay source is alkaline. However, if the subsurface soil is acidic this will compound existing surface soil acidity. Added clay will also increase the buffering capacity of the surface soil, which can help to reduce the impact of soil acidification, though not addressing the problem directly.
Strategies for the management of soil water repellence can have benefits for weed control, but also some negative impacts.
Blanket application of wetting agent as a mitigation strategy for soil water repellence will have benefits for weed control since it improves synchronous germination of weeds, aiding efficacy of herbicides. Added to this, wetting agents can improve the effectiveness of herbicides by acting as surfactants, aiding the entry of soil-applied herbicides into soil.
Soil inversion to alleviate soil water repellence has added benefits for the control of weeds. Buried topsoil reduces the seedbank of weeds, placing them too deep to enable later germination. This is especially important if the seeds are from weed plants that are resistant to herbicides. Complete soil inversion is required to achieve optimum burial of weed seeds, so complete soil inversion with a mouldboard plough is better at controlling weeds than deep soil mixing with a rotary spader, although the latter will provide some benefit for weed control.
As with wetting agents, clay spreading or delving can have an indirect benefit of controlling weeds due to more-even germination of weeds and improved efficacy and timing of control techniques. Management of soil acidity has been found to reduce the weed seed bank due to increased efficacy of herbicides and greater competitiveness of crop plants.
Where soil water repellence has been effectively managed, plants are more likely to have strong early root growth and less water and nutrient stress, making damage from soilborne diseases and nematode pests less likely. Some benefits for the control of pests and diseases may result from strategies to manage soil water repellence. Wetting agents can be used as a carrier for cheap and efficient delivery of fungicides. A reduction in leaf disease pathogens such as Septoria spp. has been measured after soil inversion, compared with an untreated control, possibly due to burial of pathogen spores.
However, it is possible that surfactants that enable connectivity of water films in soil pores and alleviate water repellence may also assist the movement of pathogens through soil. This could aid in the movement of plant parasitic nematodes (e.g. Pratylenchus spp.) toward plant roots and may decrease water stress influences on their life cycle.
On-row sowing to mitigate soil water repellence increases fertility below the crop rows since nutrients, organic matter and associated soil biota are concentrated in the same row each year.
Furrow sowing and the application of wetting agents increase the risk of nutrients leaching beyond the root zone. The preferential flow associated with furrows may concentrate soluble nutrients, which may then leach deeper into soil.
The adoption of narrow furrows where stubble is retained decreases the risk of preferential water flow. Adding a band of wetting agent can make small furrows more efficient for water harvesting.
Blanket application of wetting agents can also increase the risk of deep drainage and leaching associated with a decrease in the surface tension of the water and results in lower water and nutrient uptake by plants.
Claying may add essential plant nutrients to the topsoil. Clay-rich subsurface soil may increase the levels of potassium and sodium in the clayed topsoil as well as increasing water holding capacity, soil stability, and soil organic carbon. Subsurface soil used for claying may also have high levels of boron, be sodic or acidic, so it is essential to test the soil prior to spreading or delving.
Waxes within organic matter cause water repellence. In soils susceptible to water (e.g. sandy textured soils), and increase in soil organic matter can lead to higher likelihood and severity of water repellence.
Water repellent topsoil (that is concentrated in organic matter) buried by soil inversion, stays wetter for longer than if remained at the surface. Burial of the water repellent topsoil can improve plant access to nutrients due to extended periods of biological activity and subsequent breakdown of the organic matter.
On water repellent soils, low disturbance systems such as no-tillage cropping have demonstrated benefits for crop establishment and grain yield due to the preservation of remnant roots which behave as preferential pathways for water entry.
Collins S, Mwenda G, Wilkinson C, Hüberli D, Kelly S, Gajda K, Reynolds C, Kupsch M, Wickramarachchi K, Hunter H, Zaicou-Kunesch C, van Burgel A, Linsell K, Davies S (2021). Mechanical soil amelioration alters soil biology, soilborne pathogen and nematode pests of cereal crops: what are the implications? Paper presented at GRDC Grains Research Updates, Perth. [online]
Roper MM, Davies SL, Blackwell PS, Hall DJM, Bakker DM, Jongepier R, Ward PR (2015). Management options for water-repellent soils in Australian dryland agriculture. Soil Research 53(7), 786-806. https://doi.org/10.1071/SR14330
Economic considerations
Unless the area of the farm affected by water repellence is relatively small it is generally more profitable to first adopt improved furrow sowing techniques. Progressive amelioration can then be undertaken in areas where responses are likely to be high and sustained.
Soil mixing or inversion provides long-term and reliable benefits for most repellent soil. In most situations break-even returns are achieved within two years.
Significantly higher benefits are generated when strategic deep tillage and other amelioration options are combined to address limiting constraints within a soil. This may include use of more than one strategic deep tillage method (e.g. deep ripping as well soil mixing/inversion) with incorporation of ameliorants such as fertilisers, clay, lime, and/or organic matter.
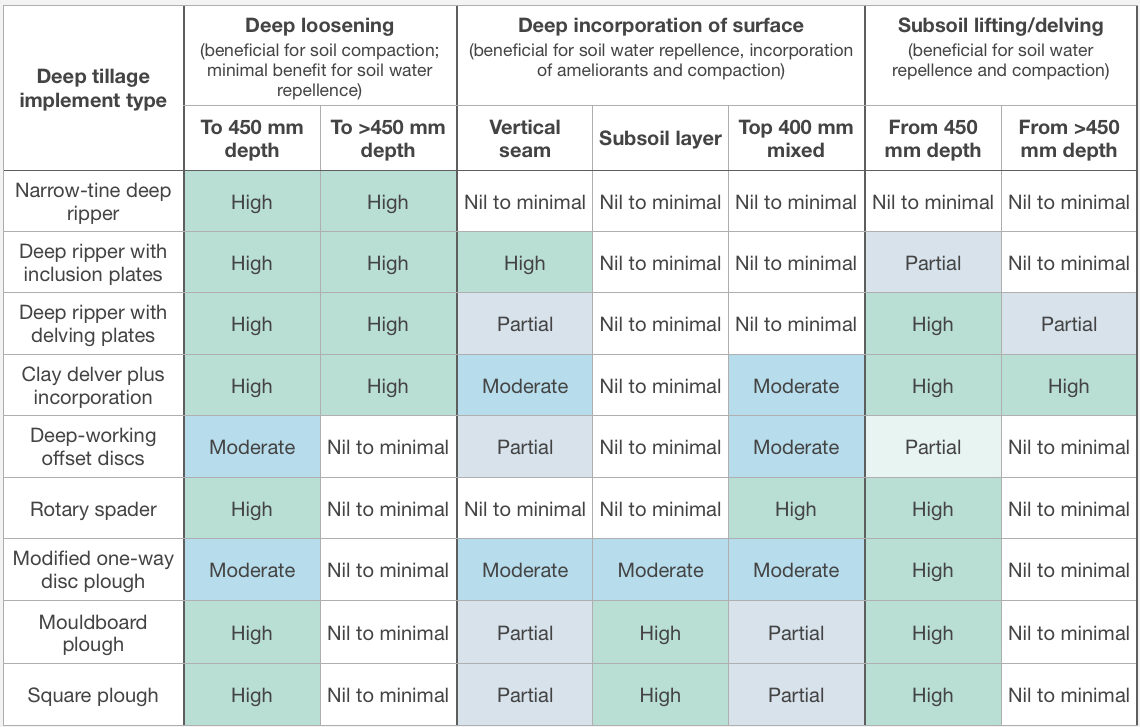
Davies SL, Armstrong RD, Macdonald L, Condon JR, Petersen E (2019). Chapter 8. Soil constraints: a role for strategic deep tillage. In: J Pratley and J Kirkegaard (Eds) Australian Agriculture in 2020: from conservation to automation. Agronomy Australia and Charles Sturt University. pp 117–135. [online]
Davies S, Betti G, Edwards T, McDonald G, Hall D, Anderson G, Scanlan C, Reynolds C, Walker J, Poulish G, Ward P, Krishnamurthy P, Micin S, Kerr R, Roper M, Boyes T (2019). Ten years of managing water repellent soils research in Western Australia – a review of current progress and future opportunities. Paper presented at 2019 GRDC Grains Research Updates, Perth. [online]
Parker W, Isbister B (2020). Subsoil compaction management: outcomes of five years research in WA. Paper presented at GRDC Grains Research Updates, Perth. [online]
Scanlan CA, Davies SL (2019). Soil mixing and redistribution by strategic deep tillage in a sandy soil. Soil and Tillage Research 185: 139–145. https://doi.org/10.1016/j.still.2018.09.008
Ucgul M, Saunders C, Desbiolles JMA, Davies SL, Parker W (2019). Improving the effectiveness of soil amelioration by optimising soil machine interaction. Paper presented at GRDC Grains Research Updates, Perth. [online]
Page references and acknowledgements
Material on this page adapted from:
- Davies S, Fisher J, Roper M and Murphy D (2022). Soil Quality: 7 Soil Water Repellence. SoilsWest, Perth, Western Australia. [Access]
- Pluske W, Boggs G and Leopold M (2018). Soil Quality: 2 Integrated Soil Management. SoilsWest, Perth, Western Australia. [Access]
Last updated August 2024.