Soil chemistry strongly influences soil structure, root growth, and plant health.
Crop and pasture production can be limited by nutrient deficiencies and toxicities, as well as by adverse values of other soil properties such as pH, mineralogy, cation exchange capacity, and organic matter content.
In most cases, conditions that restrict plant growth can be detected by soil testing – which can be guided by longer term productive capacity, historical records and on-farm observation. Regular sampling and testing provide land managers with the information to make decisions regarding soil management to optimise production and minimise environmental risk.
Ultimately, the goal should be to have a clear nutrient management strategy for each part of the farm that aims to maximise profit and environmental sustainability over the chosen timescale.
When is the right time to test soil?
Whilst there is no wrong time to test soil, farmers may want to test just prior to a cropping program to ensure sufficient nutrients are available for the incoming crop. Collecting a representative sample and considering depth of sampling is important in determining what nutrients are available to a growing crop or pasture.
Testing soil at the same time each year allows a comparison of results between years and builds a clear profile of soil nutrient levels over time.
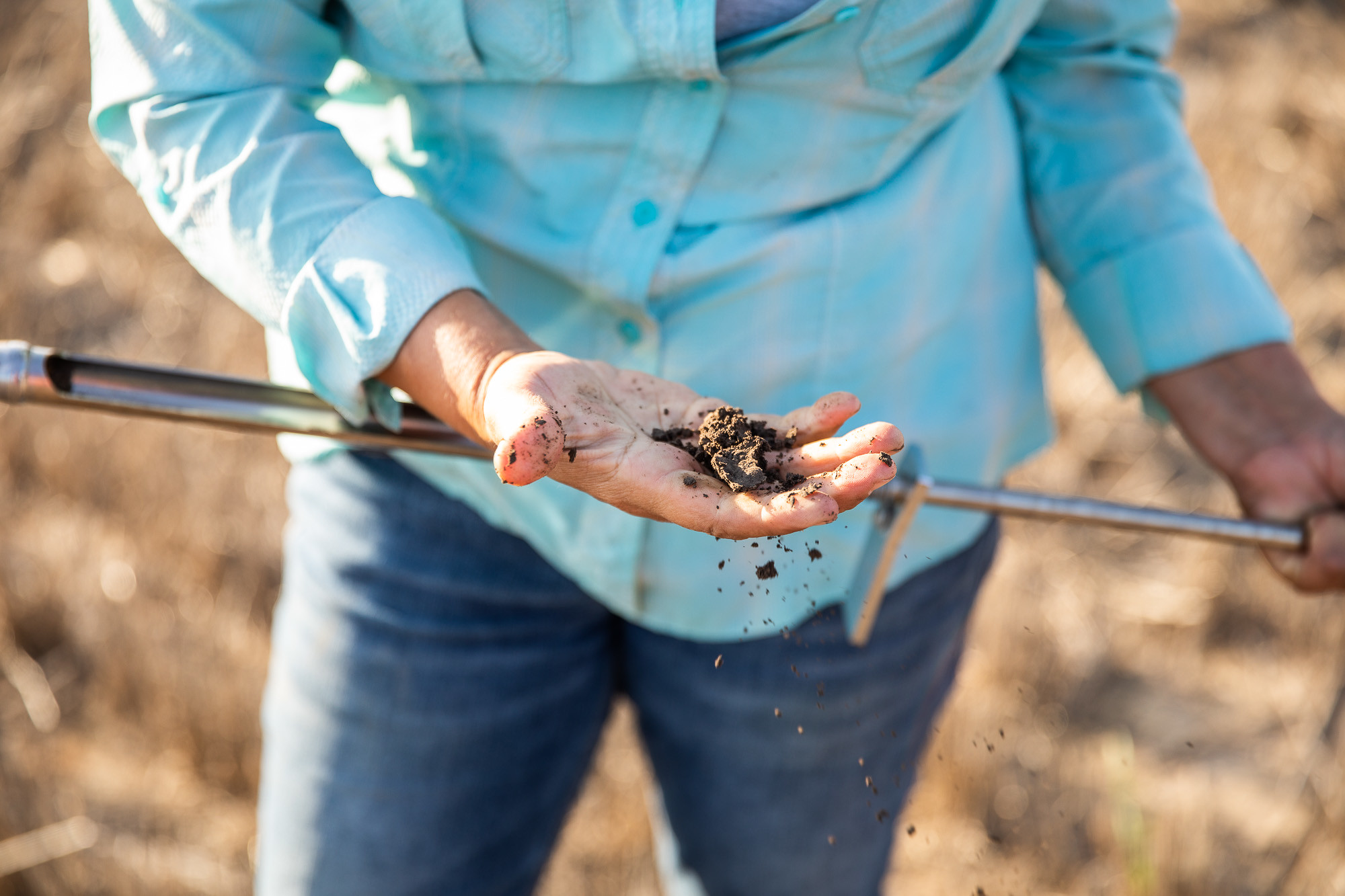
Growers and advisors should be aware of the method of analysis used in soil tests, which should always be stated. Analytical methods, even for a simple test like soil pH (i.e. pH in water, or 0.01M CaCl2), can influence the interpretation of results which depends on calibration of the test data with field measurements of crop yield and response for appropriate field conditions (e.g. soil, climate, crop species and variety).
Methods may have been developed and calibrated without local validation (potentially on different crops or soil types), and this is often an area where there is significant variability in advice, with transparency of recommendations potentially blurred by ‘commercial-in-confidence’ issues.
If in doubt about a specific recommendation, farmers should seek further advice.
Recording and maintaining good records of soil tests and fertiliser decisions is essential for planning and evaluating the performance of nutrient management.
Regular soil testing and analysis will aid in building up a site history which shows trends through time, but care must be taken in ensuring that analyses done from year to year are undertaken using the same methods for analysis, to reduce confusion associated with different analytical techniques or sample handling.
When comparing different sites or investigating trends in soil fertility over time, it is important to use the same analysis method. For example, pH determined by adding water to soil will typically be higher than if pH of the same soil were determined by adding a solution of calcium chloride. While soil analysis results may appear to be very precise in the report, these only relate to the sample of soil as submitted and depend on how representative that sample is of a paddock, or larger area.
Interpretation needs to be much broader to understand trends in, and developing recommendations for, the whole area from which the (generally small) sample was taken. Since the bulked sample is used to represent an average for a much larger area, the reported soil test data should only be treated as indicative rather than as absolutely precise. Thus, small changes from one sampling date to the next do not necessarily indicate a developing trend or a need to change current management practices. This is where an experienced agronomist, regional trials and local knowledge can help by ensuring that the data are interpreted realistically.
Nitrogen
Mitigation of increasing input costs and potential risk to the environment can be achieved by more efficient use of both organic and inorganic sources of nitrogen. Soil testing is useful in quantifying nitrogen potentially available for growing plants.
Soil organic matter turns over at a rate of about 2% annually, with changes in the net amount of nitrogen available to plants driven by biological processes occurring during the release (mineralisation) and subsequent fate (e.g. nitrification) of organic nitrogen.
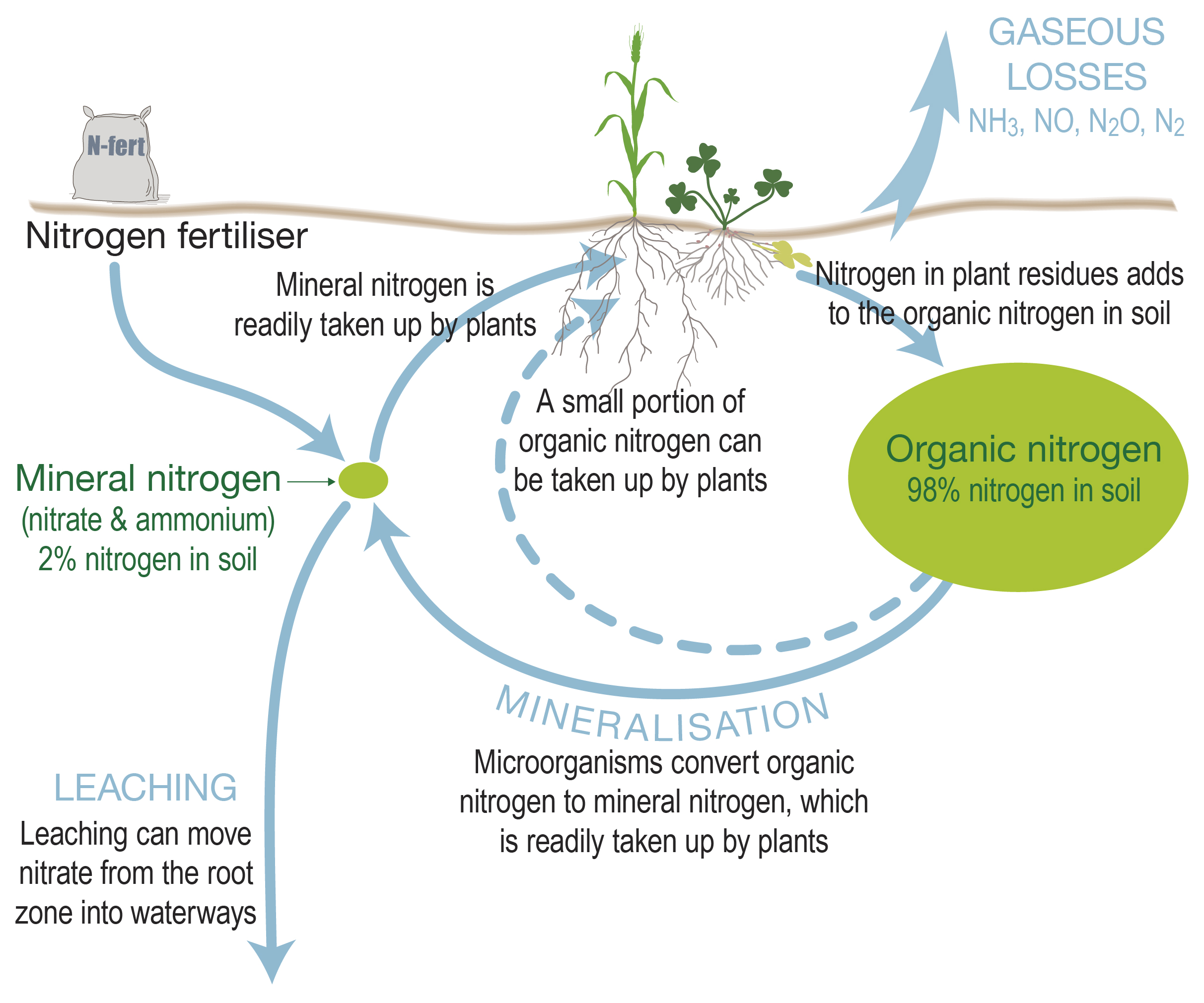
Soil mineral nitrogen can change rapidly in response to climate and management. Soil testing helps to predict the requirement for fertiliser applications to meet target yield and grain protein.
While soil testing is most often conducted prior to sowing, deep soil nitrogen testing during crop tillering can help managers understand the nitrogen pool available to plants, taking into consideration mineralisation of organic matter from soil and /or crop residues.
Soil analysis (measure) | How is it measured? | Units of measurement |
---|---|---|
Total nitrogen (Dumas combustion; also referred to as LECO) | Combustion of soil samples at 950°C and nitrogen is measured in the gases produced. | % of soil weight that is nitrogen. |
Mineral (inorganic) nitrogen is a measure of the amount of nitrogen in a plant-available form at the time of sampling. This test is used in decision support models to estimate total supply of plant-available nitrogen during a growing season. | Mixing of soil with a potassium chloride solution to extract nitrogen in the form of nitrate and ammonium. | Measured in mg/kg (milligrams of nitrogen per kilogram of soil; equivalent to ppm or parts per million). |
Potentially-mineralisable nitrogen estimates the amount of nitrogen that could potentially be mineralised in a growing season. | Ammonium-nitrogen is measured using the method for mineral nitrogen above at two timepoints: 1. Sample received at laboratory; 2. After seven days anaerobic incubation. Potentially-mineralisable nitrogen is calculated as the difference between these two measurements. | Measured in mg/kg as above. |
Phosphorus
Factors influencing the balance of phosphorus (P) in the soil involve a combination of the chemical nature of phosphorus forms along with their degree of stability and binding energy, microbial activity, clay mineralogy, intensity of land-use and management, and phosphorus inputs.
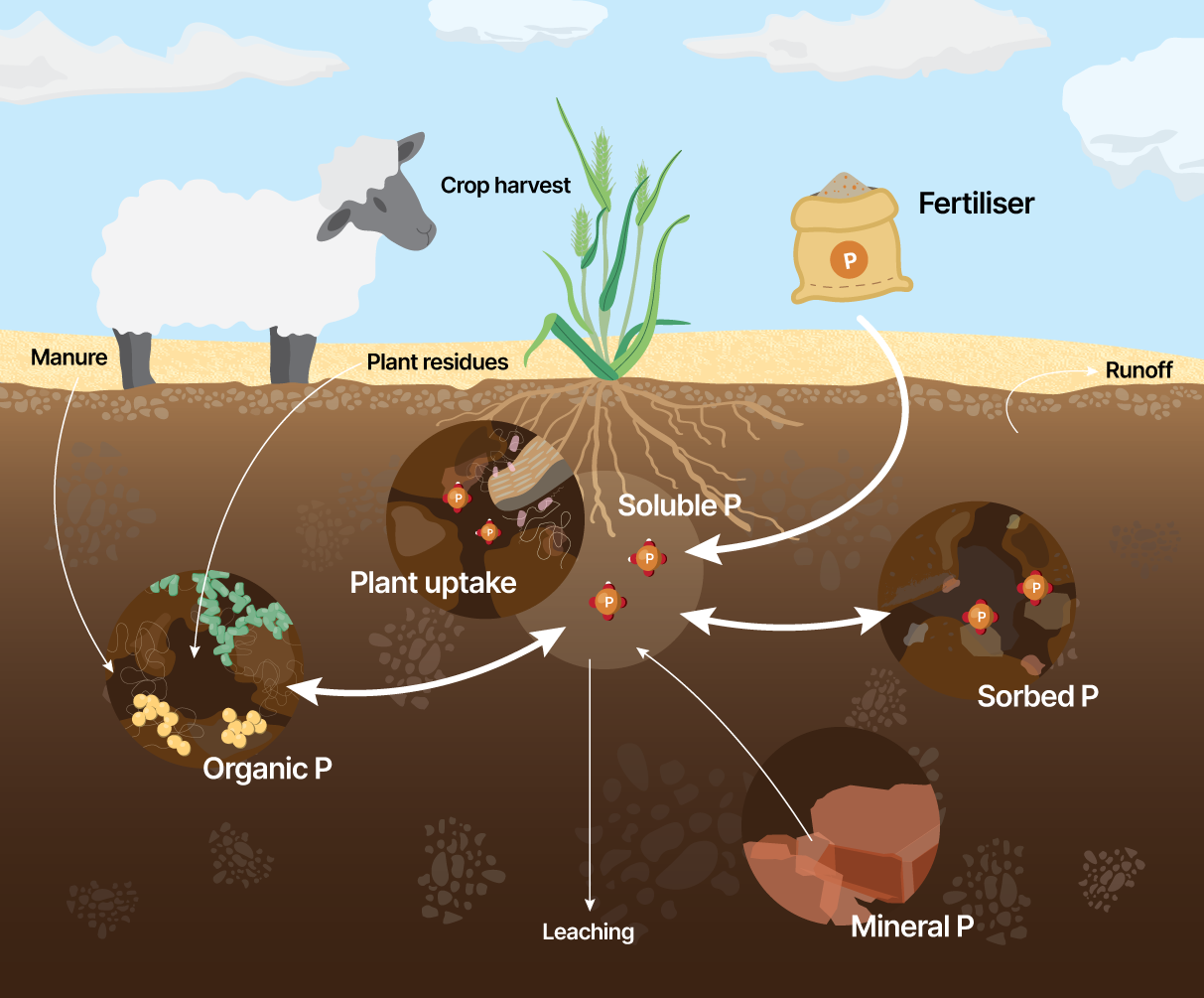
Soil analysis (measure) | How is it measured? | Units of measurement |
---|---|---|
Colwell-P measures plant-available phosphorus in soil. Decision support models predict the crop or pasture yield response to phosphorus fertiliser based upon plant-available phosphorus and a soil test calibration curve. | Mixing with a sodium bicarbonate solution for 16 hours to extract phosphorus that sorbed to soil. | Measured in mg/kg (milligrams of nitrogen per kilogram of soil; equivalent to ppm or parts per million). |
Olsen-P measures plant-available phosphorus in soil. Decision support models predict the crop or pasture yield response to phosphorus fertiliser based upon plant-available phosphorus and a soil test calibration curve. | Mixing with a sodium bicarbonate solution for 30 minutes to extract phosphorus that sorbed to soil. | Measured in mg/kg as above. |
DGT-P measures plant-available phosphorus in soil. Decision support models predict the crop or pasture yield response to phosphorus fertiliser based upon plant-available phosphorus and a soil test calibration curve. | A device that has a diffusive gel attached to an iron oxide film that is placed in contact with a saturated soil sample for 24 hours. | µg/L (microgram per litre) |
Phosphorus buffering index (PBI) quantifies that phosphorus buffering of the soil, which can be used with other soil phosphorus tests (such as Colwell-P) to estimate to level of phosphorus sufficiency of the soil. This provides an estimate of the capacity of the soil to retain phosphorus prior to any phosphorus addition. | Soil is mixed with a 100 mg P/L solution for 17 hours at a ratio of 10:1. The PBI is calculated from the amount of phosphorus sorbed from the solution at the end of the extraction, and Colwell-P or Olsen-P to account for phosphorus already sorbed to the soil. | |
Calcium chloride extractable phosphorus is used to estimate the potential for dissolved reactive phosphorus loss due to correlation with soil solution phosphorus. | Soil extracted with a 0.005-0.01M calcium chloride solution for 16 hours. | mg/kg or µg/kg |
Phosphorus Environmental Risk Index (PERI) is correlated with calcium chloride extractable phosphorus and is an estimate of the potential for dissolved reactive phosphorus loss. | Calculated by dividing Colwell-P by PBI+Colwell-P. |
Potassium
Potassium deficiency is commonly observed in sandy soil, and increasingly evident in duplex and loamy soils with significant removal of potassium from soil in produce. Soil and plant tissue testing provide valuable insight into availability of potassium in the soil.
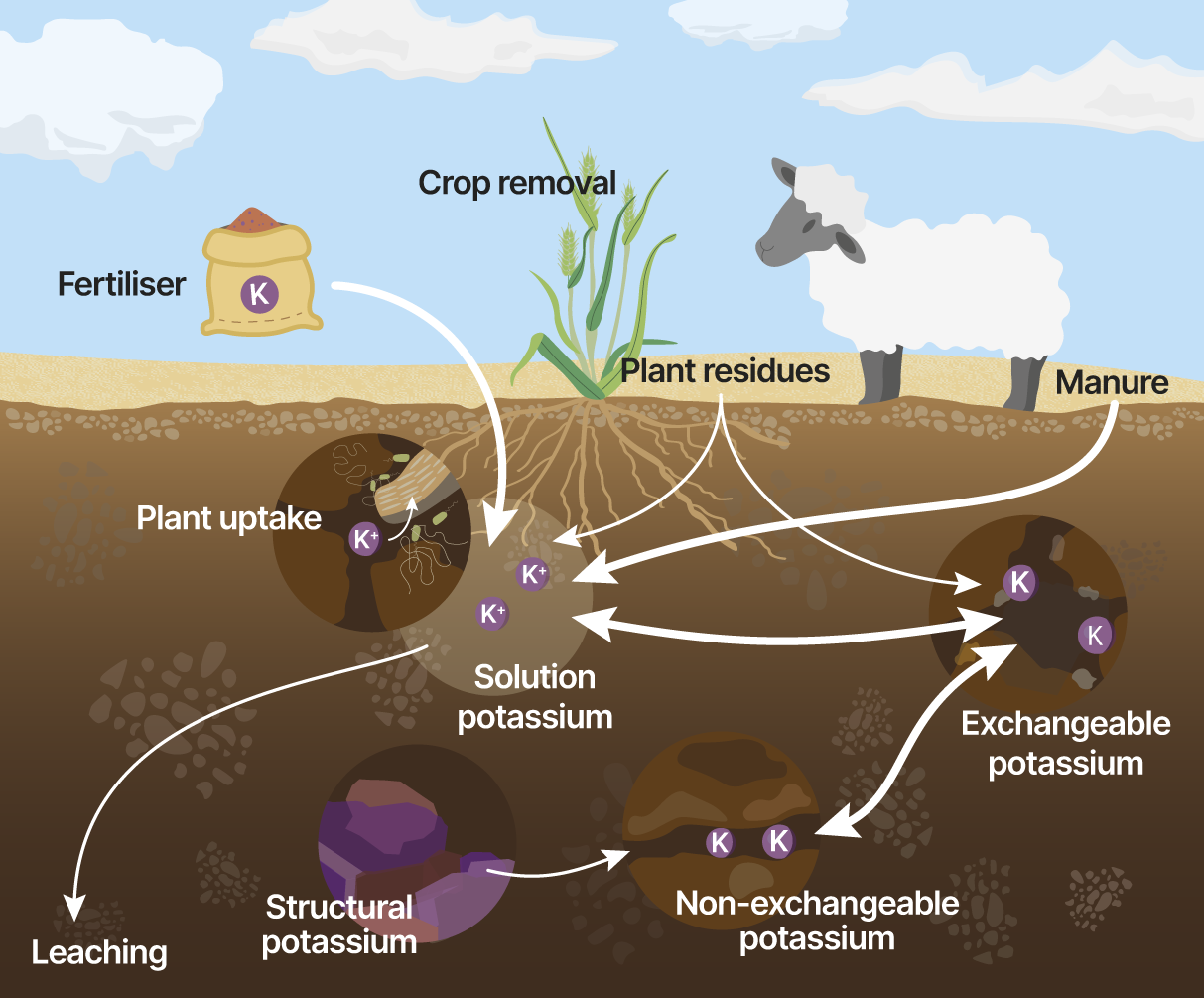
Soil analysis (measure) | How is it measured? | Units of measurement |
---|---|---|
Colwell-K measures plant-available potassium in soil. Decision support models predict the crop or pasture yield response to potassium fertiliser based upon plant-available potassium and a soil test calibration curve. | Mixing with a sodium bicarbonate solution for 16 hours to extract exchangeable potassium. | mg/kg |
Exchangeable potassium measures exchangeable potassium in soil. | An ammonium and barium chloride solution is used to extract exchangeable cations. | cmol+/kg (centimoles of charge per kilogram of soil) |
Ammonium-acetate potassium measures exchangeable potassium in soil. | An ammonium acetate solution extracts exchangeable cations. | cmol+/kg (centimoles of charge per kilogram of soil) |
Sulfur
The broadscale adoption of canola as a profitable break crop in the south-western agricultural region of Western Australia, has highlighted the increased requirement for sulfur in farming systems. Soil mineralisation, recycling of crop residues, removal in grain and leaching are critical soil processes determining the levels of plant-available sulfur during the growing season.
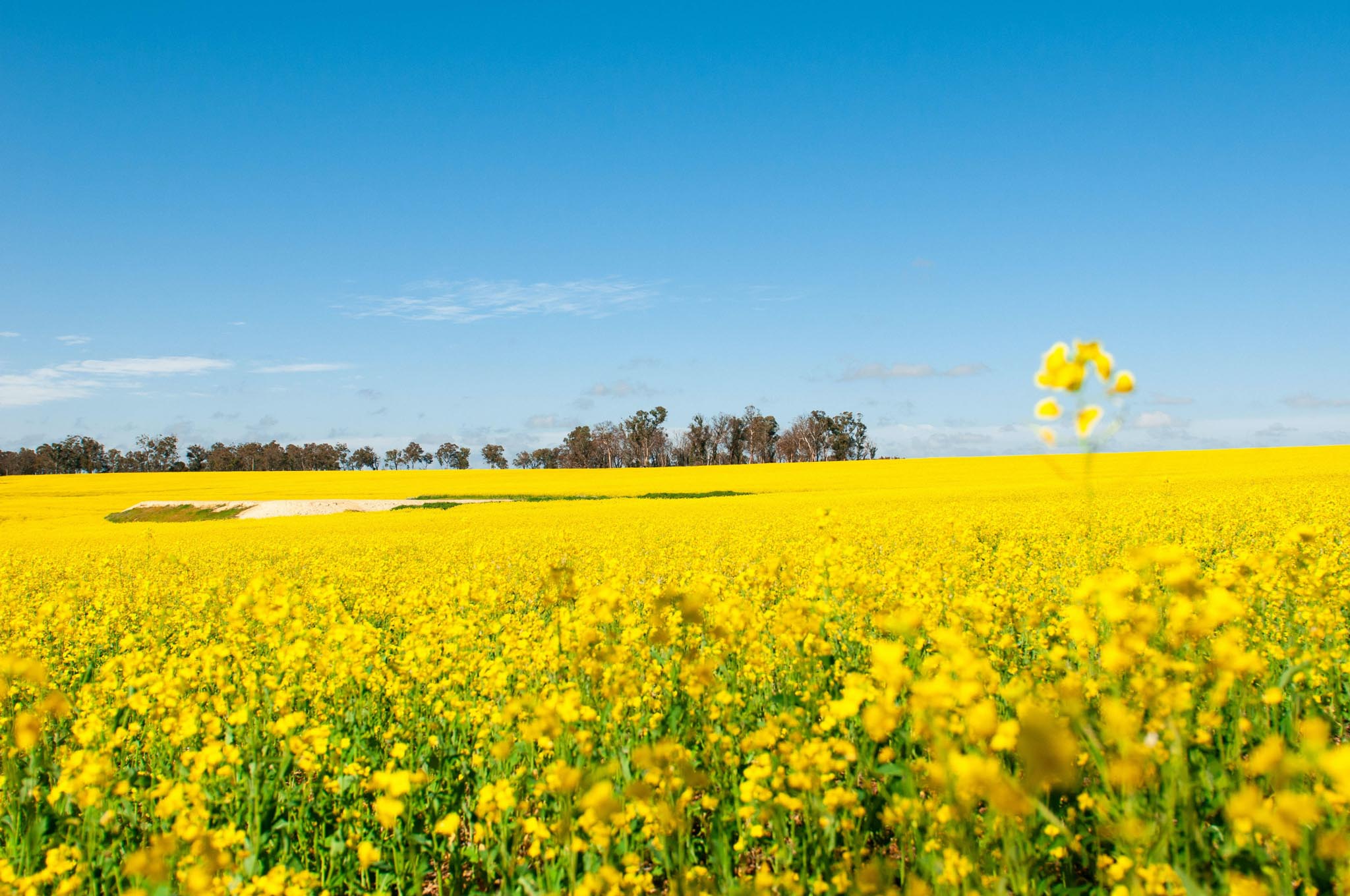
Soil analysis (measure) | How is it measured? | Units of measurement |
---|---|---|
KCl-40-S measures plant-available sulfur in soil. Decision support models predict the crop or pasture yield response to sulfur fertiliser based upon plant-available sulfur and a soil test calibration curve. | A potassium chloride solution mixed with soil sample. | mg/kg |
MCP-S measures plant-available sulfur in soil. Decision support models predict the crop or pasture yield response to sulfur fertiliser based upon plant-available sulfur and a soil test calibration curve. | A mono calcium solution mixed with soil sample. | mg/kg |
Copper
DTPA-Cu
Identifies copper deficiency in soil sample.
How is it measured?
A diethylene-triamine-penta-acetic acid (DTPA) solution mixed with soil sample.
Units of measurement
mg/kg
Manganese
DTPA-Mn
Identifies manganese deficiency in soil sample.
How is it measured?
A diethylene-triamine-penta-acetic acid (DTPA) solution mixed with soil sample.
Units of measurement
mg/kg
Zinc
DTPA-Zn
Identifies zinc deficiency in soil sample.
How is it measured?
A diethylene-triamine-penta-acetic acid (DTPA) solution mixed with soil sample.
Units of measurement
mg/kg
Boron
Boron toxicity or deficiency
Identifies boron deficiency or toxicity in soil sample.
How is it measured?
A calcium chloride solution mixed with soil sample at 90°C
Units of measurement
mg/kg
The presentation and format of the laboratory analysis report for your soil sample will vary with different providers, but it should show the results of each test, the units of measurement, and the methods used – and some also offer recommendation relating to the test results. Understanding what and how the test was conducted allows you to seek independent interpretation by qualified providers who may, for example, compare results against fertiliser response trials in your region.
Choosing a laboratory for soil chemistry testing
There are many laboratories offering a range of seemingly similar analyses for soils. There is also a wide range in methods, quality assurance and interpretation services. In an effort to establish quality control, laboratories can now be certified by the Australian Soil and Plant Analysis Council Inc (ASPAC). ASPAC conducts regular national quality assurance programs to check and improve the standard of analysis and assist in method standardisation.
The ASPAC system, along with others such as ‘Fertcare’, provides confidence through soil testing method standardisation and accreditation for the purpose of improving measurement performance and assisting with the interpretation of results.
Inevitably there is a degree of measurement uncertainty in laboratory analytical results, but the certification system imposes the need for this uncertainty to be held within acceptable limits. This should lead to less variation between laboratories and allow more meaningful interpretation of results. While there is a degree of error associated with a soil test, in-field variability and inappropriate soil sampling techniques are likely to introduce larger variations and errors than the laboratory analyses.
Farmers are recommended to seek accredited laboratories and expert advice when undertaking and interpreting soil tests. Contact your local agricultural consultant, agronomist or agribusiness for the contact details of available soil testing and support services in your region.
How to interpret soil chemistry tests
The science that underpins relationships between particular soil tests and crop and pasture responses to fertilisers and amendments has been acquired through many years of research, trials, innovation and validation.
The presentation and format of the laboratory analysis report for your soil sample will vary with different providers, but it should show the results of each test, the units of measurement, and the methods used – and some also offer recommendation relating to the test results. Understanding what and how the test was conducted allows you to seek independent interpretation by qualified providers who may, for example, compare results against fertiliser response trials in your region. Accurate interpretation of soil test results and recommendations require an in-depth (and often localised) knowledge of the factors that link the particular test with likely responsiveness.
Soil test critical values for individual nutrients are often derived according to relative yield (something like 95% of the maximum), although the benefit to cost ratio for each nutrient can affect its recommended rate to some extent. Below we provide an example of building a fertiliser recommendation model from soil tests.
Building a fertiliser recommendation model from soil tests
Most fertiliser recommendation models contain two components:
- Prediction of yield loss where no fertiliser is applied
- Prediction of yield response to increasing rates of fertiliser application
Predicted grain yield loss where no fertiliser is applied, is typically calculated using current soil test values and a soil test calibration curve to determine potential loss from the maximal yield level. Predictions can be improved where other variables to improve the accuracy of any potential yield loss, such as the previous crop, organic carbon, or phosphorus buffering index are included.
Models used to predict a nutrient rate-response are typically built by modifying parameters to provide the best fit to a broader database of nutrient rate-response data from field trials. The predicted rate-response is then used to predict the economic response based upon grain and fertiliser prices.
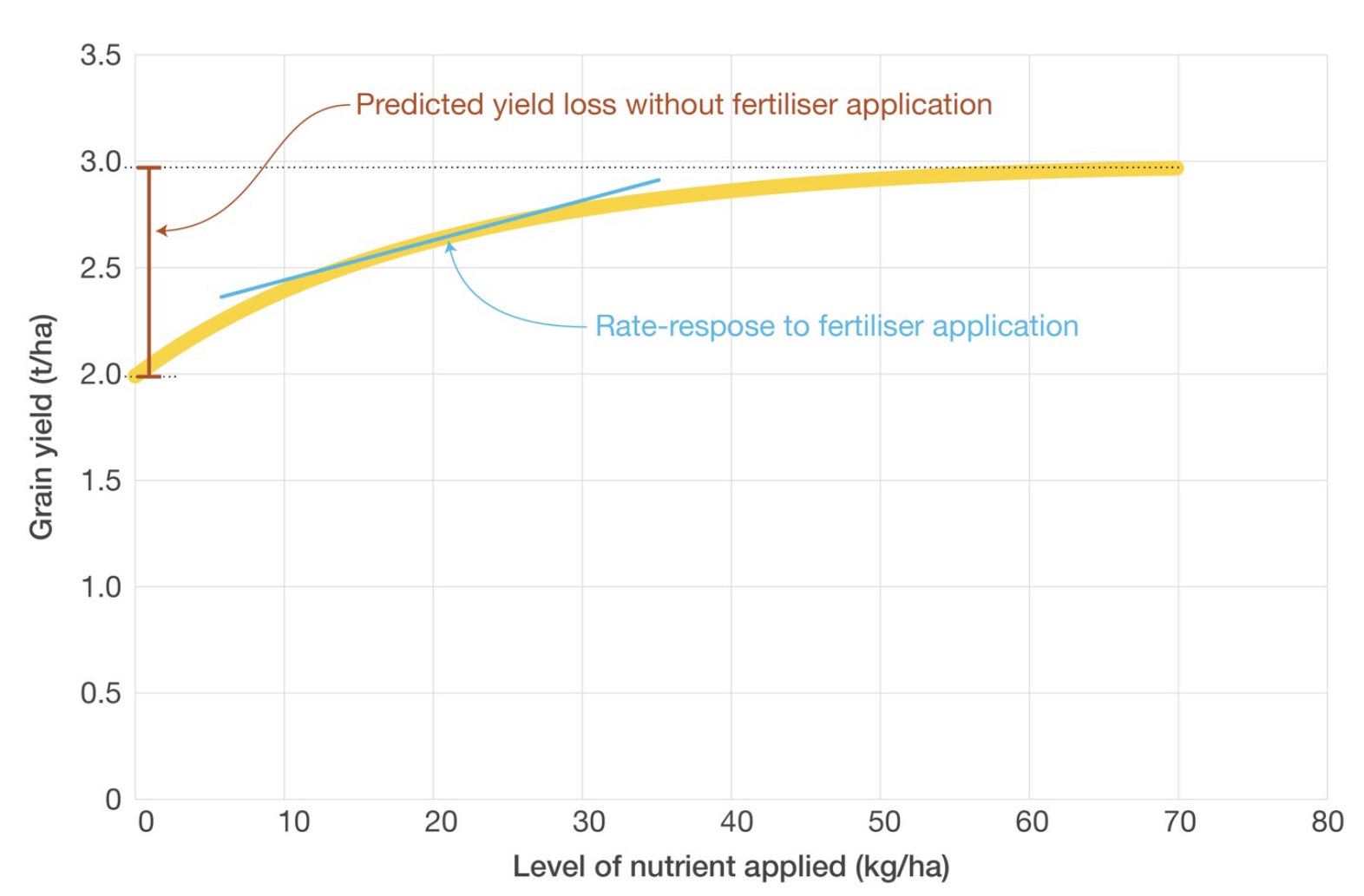
Soil test calibration curves
Soil test calibration curves are used to calculate the level of yield loss at a known soil test value, or to calculate the soil test value required for 90% relative yield (critical values).
Soil test calibration curves are used in fertiliser recommendation models to calculate predicted yield loss based on the soil test value and an estimate of potential yield.
Critical values are calculated as the soil test value where a relative yield of 90 or 95% is expected. A threshold of 90 to 95% relative yield is used because it is an indicator of economic responses – where soil test values are above this level, an economic benefit from fertiliser application is unlikely.
More recently, critical ranges were introduced to quantify the statistical confidence for the critical level, which as quantified as a confidence interval. In the figure below, there is a 90% confidence that the soil test value that will provide a relative yield of 90% is between 18 and 25 mg/kg.
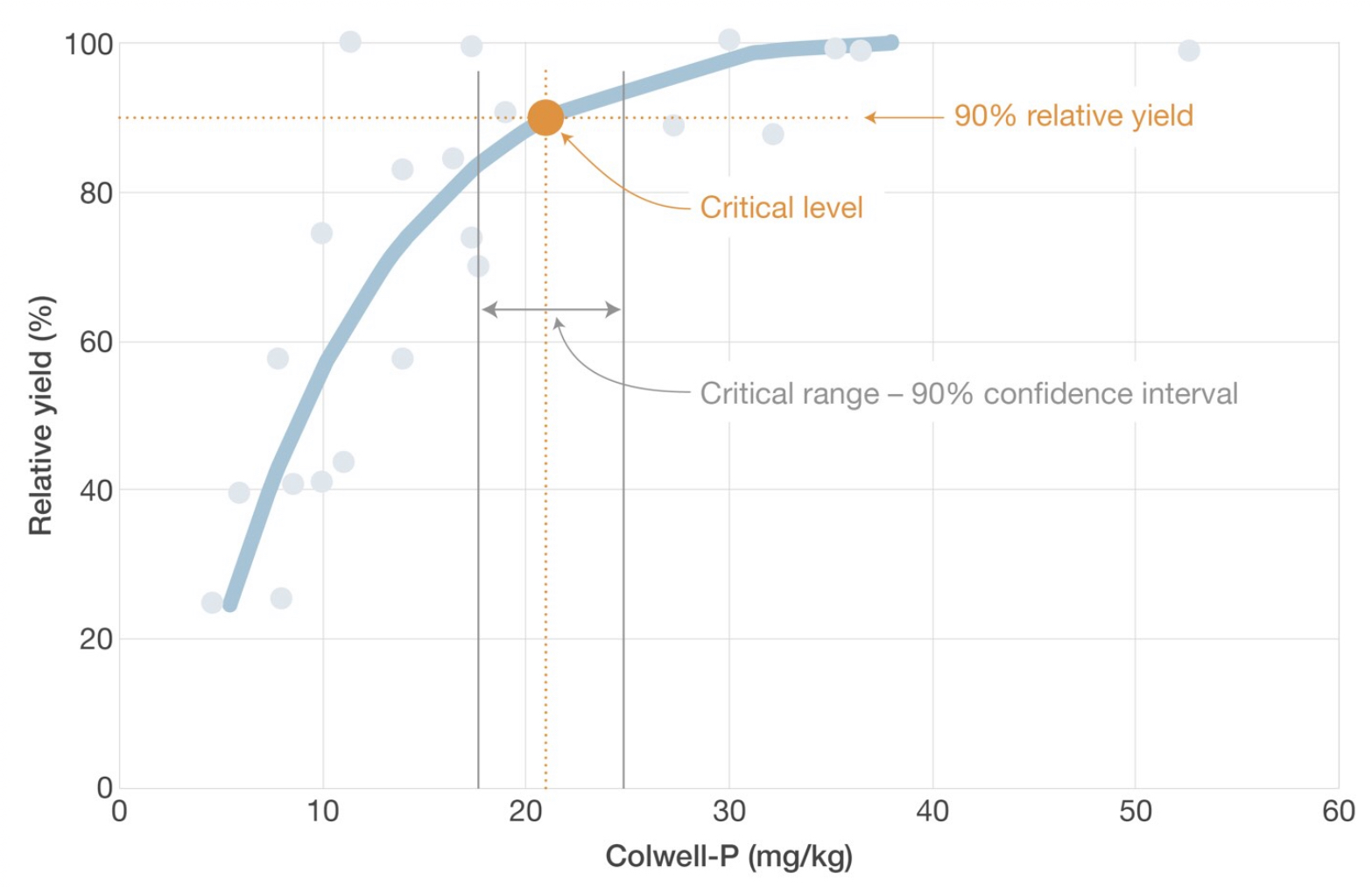
Critical soil test levels
Critical levels for soil nutrient concentrations are frequently used in nutrient management to identify a threshold where a change in yield-response to fertiliser would occur. Critical levels are determined using soil test calibration curves by calculating the soil test level at which 90 to 95% of maximum yield occurs. When soil test levels are above the critical level, on average a yield response of less than 10% would be expected. More recent analysis of soil test calibration curves in Australia has accounted for the variability in this relationship by providing a range (i.e. the critical range) where 90% of maximum yield is most likely to occur.
In some cases, several soil test calibration curves are required for a nutrient. For example, different soil type calibration curves were identified for different soil types for response to phosphorus fertiliser in wheat; the critical range (for 90% of relative yield) for deep grey sands was 16–25 milligrams of phosphorus per kilogram (measured as Colwell-P) and was 21 to 30 milligrams per kilogram for sandy earths and loamy earths. For pastures, soil test calibration curves for Colwell-P were differentiated by phosphorus buffering index, and for Colwell-K were differentiated by soil texture. In pastures for example, for soil with a low phosphorus buffering index of less than 5, the critical Colwell-P to achieve 90% relative yield is 8 milligrams of phosphorus per kilogram compared to a critical Colwell-P of 40 milligrams of phosphorus per kilogram when phosphorus buffering index is 500. For potassium in a sandy textured soil, the critical Colwell-K is 96 milligrams of potassium per kilogram compared to 121 for a clay loam textured soil to achieve 90% of relative yield.
The calculator at the link below can assist to determine critical levels for phosphorus, potassium and sulphur for high rainfall pastures.
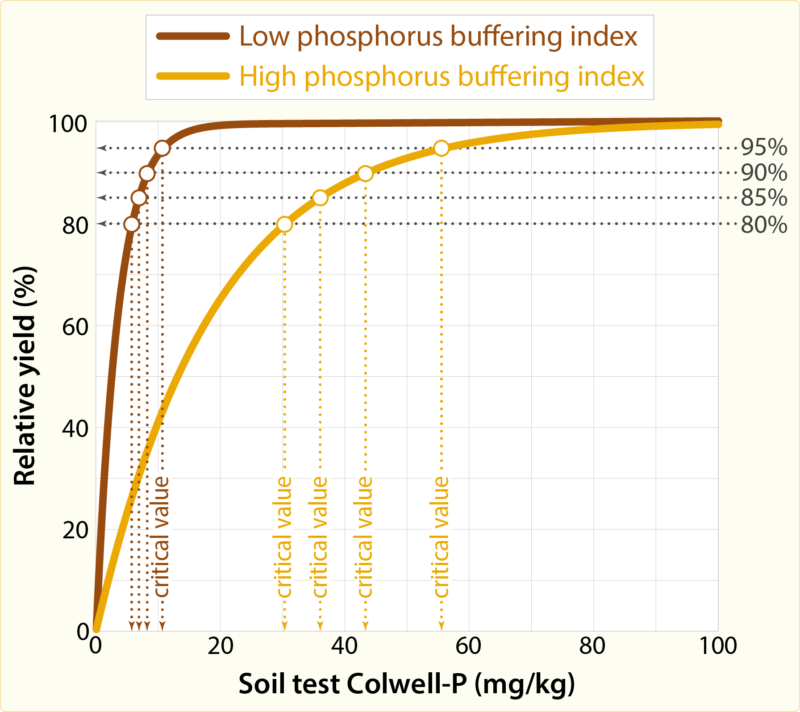
The effect of nutrient concentration on plant growth depends on how the supply of that nutrient relates to plant requirements and tolerance. In the south-western agricultural region, most of our attention is focussed on overcoming nutrient deficiencies, but there are scenarios where nutrient toxicities can occur. For example, boron toxicity can occur where boron concentration in the subsoil is above 5 milligrams per kilogram, which is associated with soil with alkaline, sodic subsoil in mallee regions in the south-eastern wheatbelt. Barley and field peas are the most susceptible crops to boron toxicity.
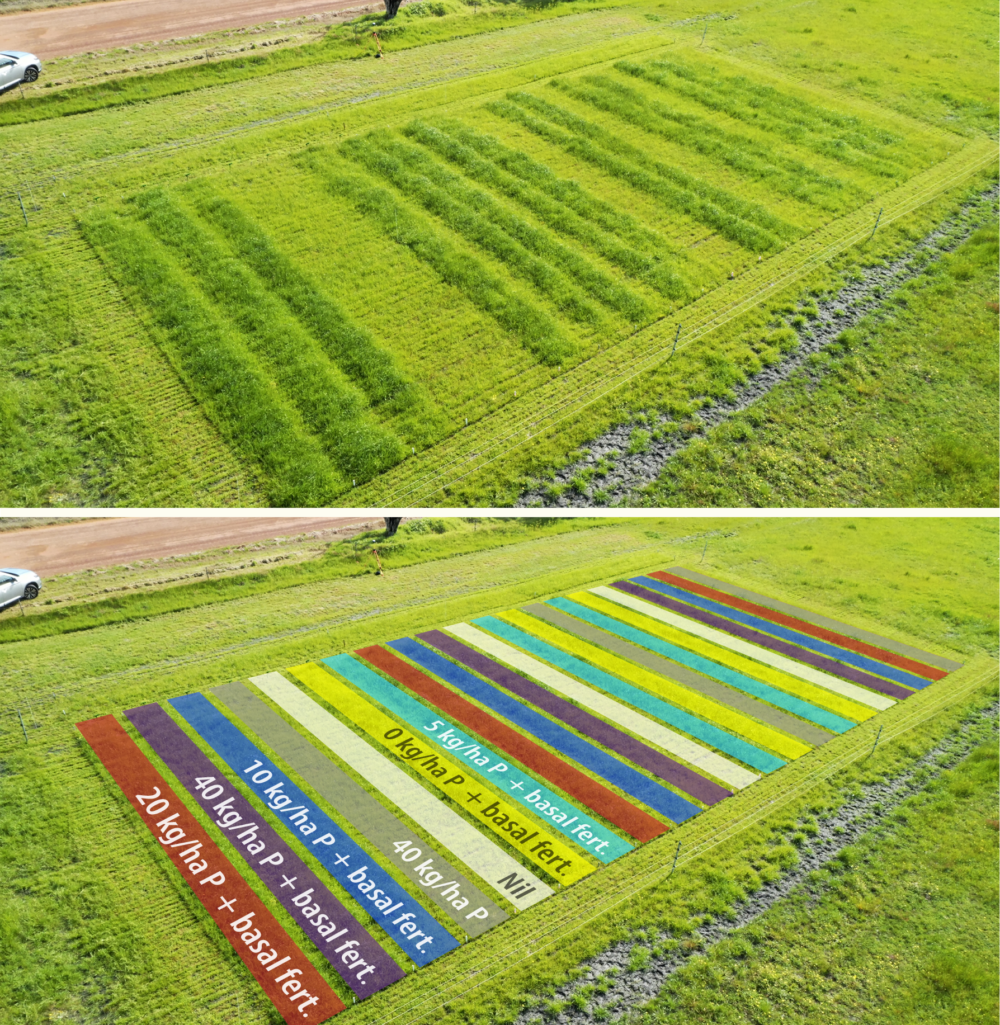
PAGE REFERENCES AND ACKNOWLEDGEMENTS
Material on this page adapted from:
- Hoyle FC (2007). Soil Health Knowledge Bank.
- Scanlan C, Weaver D, Bell R, Borrett R and Cheng M (2023). Soil Quality: 10 Plant Nutrition. SoilsWest, Perth, Western Australia. [Access]
Last updated July 2024.